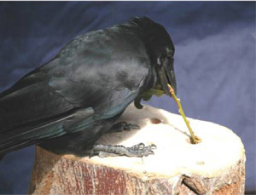
Figure 1. A New Caledonian crow
uses a stick tool to extract mealworms from a drilled
log in the Oxford laboratory. Photo: Lucas Bluff
(reprinted with permission). |
|
Our group has studied
tool-oriented behaviour (TOB) in New Caledonian crows (Corvus
moneduloides) for the past six years (Figure 1). This followed the
first systematic documentation of wild New Caledonian crow behaviour by
Gavin Hunt and associates from Auckland University (Hunt, 1996, 2000a;
Hunt, Corballis, & Gray, 2001; Hunt & Gray, 2003). Here we present a
progress report that focuses on experimental studies with captive crows
in Oxford. We discuss the results of our experiments with reference both
to the field data collected by our New Zealand-based colleagues and to
theoretical issues faced by other researchers in comparative cognition.
We address—but do not solve—the epistemological difficulties inherent in
attempts to uncover aspects of physical understanding and reasoning in
non-human species. Our broad perspective is that while tool-related
behaviour is not necessarily associated with unusually sophisticated
cognition, it is likely to be unusually revealing about the cognitive
processes and the level of understanding involved in animals’
manipulation of physical objects.
Uniquely amongst
non-mammals, New Caledonian crows make and use a number of distinct tool
types in the wild and in captivity (Figure 2), some of which involve
considerable processing of the raw materials (Hunt, 1996; Hunt & Gray,
2004a, 2004b). Tool use and manufacture by animals has attracted
considerable interest from scientists and the general public, probably
because of its rarity and its apparent association with the human
lineage. Whilst it is now widely accepted that tool use per se is
not indicative of unusual intelligence (e.g., Alcock, 1972; Beck, 1980,
1986; Hall, 1963; Hansell, 1987), the remarkable complexity of New
Caledonian crows’ natural TOB has often led observers (lay people as
well as scholars) to assume that this species may possess exceptionally
advanced cognitive abilities. However, such first impressions should be
treated as working hypotheses at best, as the motor complexity of a
behaviour offers no guide to its underlying cognitive complexity: There
are many examples of complex architecture—typically in the form of
nests—throughout the animal kingdom (Hansell, 2005), none of which are
thought to require generally elevated cognitive abilities.

Figure 2. New Caledonian crows
make and use a range of tool types. The tools shown here
were made from: (a) twigs; (b) Pandanus leaves; (c) leaf
stems and cardboard; and (d) moulted crow feathers. The
Pandanus leaf tools and counterparts were collected by
Gavin Hunt in New Caledonia, the rest were made in
captivity in Oxford. From Figure 26.1, page 518,
"Cognitive Adaptations for Tool-related Behaviour in New
Caledonian Crows," by A. Kacelnik, J. Chappell, A. A. S.
Weir, and B. Kenward. In Comparative Cognition:
Experimental Explorations of Animal Intelligence (eds.
Wasserman, E.A., & Zentall, T.R.), pp. 515-528.
Copyright 2006 by Oxford University Press. Adapted with
permission. |
|
Natural behaviour arises
through the interaction of the genetic endowment with a number of
ontogenetic processes that include trial-and-error, associative and
social learning, and possibly reasoning. It is axiomatic that the
contribution of each of these, and especially of ‘higher’ cognitive
processes such as reasoning or insight, cannot be reliably inferred from
observing spontaneous behaviour of wild animals without experimental
investigation. Even when experimentation is possible, these processes
are fiendishly elusive because virtually everything an adult animal does
is affected by previous associative learning and generalisation. This
difficulty is, of course, the strongest justification for the
behaviourists’ reluctance to even invoke the possibility of entities
such as reasoning or understanding, and we do have sympathy with this
argument by parsimony. However, the complete learning history of
anything but newborn individuals is never fully known. Therefore,
although attributing innovative problem-solving to a hypothetical
combination of reinforced learning and generalisation appears
parsimonious because the mechanisms are uncontroversial, it is in fact
just as speculative as accounts involving abstract forms of information
processing that would qualify as ‘reasoning’ or ‘understanding’.
We are fully aware that
such information-processing mechanisms are not alternatives to
associative learning but may act in concert to produce innovative
behaviour. However, it would seem agreeable to most that, when observing
a behavioural innovation, the more contrived the required generalisation
from previously reinforced behaviour, the more plausible it is to invoke
the existence of alternative mechanisms involving higher level concepts
(we have made similar points elsewhere; see Weir & Kacelnik, 2006). This
is the theoretical framework in which we seek to explore the involvement
of mechanisms best defined in terms of their folk-psychological labels,
such as reasoning, understanding, and creativity, in tool-oriented
behaviour of our model species.
Although TOB is not
necessarily associated with unusual cognition, the making and using of
tools may be especially revealing from a cognitive perspective. Making
and using objects to act on other objects, such as food, may give us a
valuable glimpse of an organism’s use of abstraction or generalisation
in the physical domain. Tool use entails a behavioural richness that can
be exploited in controlled experiments where it is unlikely that the
subjects can ‘solve’ the task by deployment of a previously reinforced
behaviour or a combination of such behaviours. It is challenging to
design and interpret such experiments, but TOB offers a context in which
the opportunity for innovation is greater than in situations where
subjects are intensively trained to collect rewards using binary
manipulanda such as pecking keys or levers.
So far, we have
investigated two different aspects of New Caledonian crows’ TOB: the
epigenetic processes that lead to the
development of fully-functional TOB, and the cognitive processes
that are involved in the deployment of TOB in adult crows. While
both levels of enquiry are necessary to understand this species’
cognitive capacities, it is important to recognise that, at different
periods within a crow’s life, different mechanisms may govern the
expression of functional tool use. For example, observing the
spontaneous emergence of a non-reinforced behaviour during early
development leads us to infer the presence of a genetic propensity for
that behaviour, but this does not imply that its subsequent deployment
is unaccompanied by information-processing mechanisms that would qualify
as higher cognition. Indeed, genetic predispositions are thought to be
the building blocks through which human children learn about the
properties of objects (Lockman, 2000), yet older humans are able to
devise solutions to novel problems through processes such as reasoning.
We acknowledge that
exploring the cognition behind action, especially in the context of
physical understanding, presents major epistemological challenges,
mostly because of the difficulty in isolating situations that admit a
single cognitive account. In fact, it is inherently difficult even to
define what it means to say that someone ‘understands’ their actions: A
recent philosophical review concluded that humans describe themselves as
‘understanding’ something if they believe that they are not hampered in
reaching a relevant, currently active goal by lack of knowledge about
that ‘something’ (Overskeid, 2005). Applying such a definition based on
goals and knowledge to non-humans is not easy.
Most research to date into
the causal understanding of non-humans has been framed in terms of
whether the subjects’ behaviour is generated by associative versus
inferential (or reasoning) processes (e.g., Call, 2004; Dickinson,
2001), where the ‘understanding’ of the causal basis of something (an
event or action) is equated with the ability to infer the causal
agent. However, this debate has been hampered by a lack of precision in
the specification of what is meant by reasoning or inferring: As Penn
and Povinelli (2007) point out, the advocates for such processes tend to
formulate their arguments verbally, rather than mathematically, which
makes them almost impossible to refute. Some authors do explicitly
define higher-order reasoning—for example, as "processes [that] can be
characterized as reflective […], rule-based […], and deliberate […] and
[which] operate on conscious propositional knowledge in a controlled
(i.e., slow, effortful, conscious, and/or intentional) manner" (de
Houwer, Beckers, & Vandorpe, 2005, p. 240). However, most researchers
(including, at times, ourselves in previous publications) informally
invoke reasoning or other cognitive processes for situations where
associative learning seems insufficient to explain the subjects’
behaviour. This has led to a technical debate about the mathematical
properties of associative learning and what behaviours it can and cannot
explain (e.g., Dickinson, 2001) and whether other algorithms such as
causal Bayes nets provide a better explanation (e.g., Blaisdell, Sawa,
Leising, & Waldmann, 2006). We feel that this debate does not address
one of the most interesting questions: Are any non-human animals capable
of understanding and reasoning about causality in a qualitatively
similar way to humans?
Penn and Povinelli point
out that human causal knowledge is ‘theory-like,’ "in the sense that it
provides principled, allocentric, coherent, abstract explanations for
the unobservable causal mechanisms that govern a given domain," and
suggest that a salient feature of these theories "is that they can be
generalized freely to disparate concrete examples that share little to
no perceptually based featural similarity" (Penn & Povinelli, 2007,
p.107). Mechanisms such as associative learning are undoubtedly involved
in the process of forming these theories, but the key point is that
behaviour should be influenced by the individual’s understanding, rather
than purely by the subject’s previous reinforcement history.
The main goal in our
laboratory work with New Caledonian crows is to establish whether
‘theory-like’ causal knowledge underlies their TOB—or in other words,
whether they understand how their tools work. In spite of all the
caveats listed above, we still feel that it is helpful to distinguish
between behaviour shaped by known or easily conceived experiences of
reinforcement (i.e., trial-and-error learning) and behaviour which
appears to result from an abstract process of inference.
It is worth noting that the
methods typically used to investigate cognition in non-verbal animals
are fundamentally different from those employed in studies with humans.
To probe adult humans’ understanding of the physics underlying their use
of a tool, one can ask them to verbalise their reasoning. This does not
prove that their actions are guided by their expressed beliefs (which
could be true or false regarding real physics), but it may tell us if
the subjects feel that they understand why or how the tool works
(Overskeid, 2005). Regardless of whether the use of the tool is
competent, and regardless of whether the theory expressed to justify
actions is correct, one can infer something about the existence and
quality of understanding (a narrative based on a superstitious belief
such as the gamblers’ fallacy is a mistaken but interesting form of
understanding). Even this limited level of enquiry is impossible with
non-human animals, so we have to rely on observing and interpreting
tool-using performance and to focus on definitions of understanding that
presuppose true beliefs. We are condemned to being over-conservative and
denying any understanding to an animal that does have a theory and
guides its behaviour by it, if this theory happens to be wrong.
Our general experimental
approach is to present our subjects with problems that, while being
within the range of situations that members of the species are inclined
to tackle, are as novel (namely as different from previous experiences
in which reinforcement for behavioural components have occurred) as
possible (similar to the approach taken by Hauser, 1997; Povinelli,
2000; Visalberghi, 1997). If subjects possessed abstract, theory-like
explanations for how and why their tools operate, we could expect that
in most such experiments they would solve the problems within the first
few trials. In contrast, if their behavioural modification was governed
by reinforcement, on most tasks most subjects would not be immediately
successful. Each single experiment, therefore, provides limited
information regarding how much of the observed behaviour is strictly
novel, the outcome of reasoning, or both, particularly since (a) no task
in which the birds willingly participate can ever be absolutely novel,
(b) problems do not remain novel after the first trial, and (c) adult
subjects may always generalise to some extent from previous experience.
This means that, even though we transform the tasks as radically as
possible from one experiment to another, there is no discrete threshold
separating solutions produced by pure reinforcement of random behaviour
from those resulting from understanding or reasoning.
In summary, it is very
difficult to define concepts that are used intuitively in every-day
language to denote high-level cognition, let alone to investigate them
empirically in animals. Reasoning, understanding, and logical inference
are neither the opposite of genetically-channelled actions (which do not
require reinforcement), nor of associatively learnt actions (which do).
While parsimony advises against invoking these very opaque processes
when more transparent ones are available, we still believe that the
richer the number of task transformations that subjects can tolerate and
solve, the more useful it is to invoke them.
The main part of this
review consists of two broad sections distinguishing the development and
deployment of TOB. For some of our most cherished questions, such as the
extent to which New Caledonian crows can use reasoning to solve physical
problems, or what ecological and evolutionary circumstances led to the
unusual behaviour of this species, we can only offer informed
speculation. However, we do hope to show that some parts of the puzzle
are being unravelled and that there is good reason to be optimistic
about future progress, provided we advance with caution and are able to
proceed simultaneously with experimental and observational research.
Tool-oriented Behaviour in the Wild
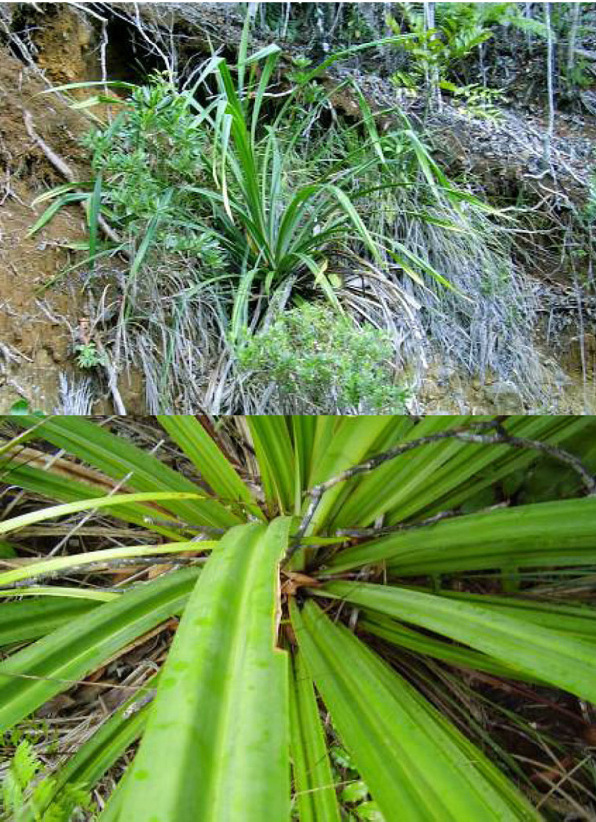
Figure 3. Some tool types are
made from the fibrous leaves of Pandanus trees (top).
Crows produce tools from leaf edges, leaving distinct
‘counterparts’ behind (bottom). These counterparts allow
researchers to infer the shape of the finished tool (see
Figure 2b). Photos: Christian Rutz (reprinted with
permission). |
|
The archipelago of New
Caledonia is situated some 1,500 km east of the Australian mainland. New
Caledonian crows are endemic to the main island of Grande Terre and one
of its sister islands, Maré, where they were introduced by humans (ca.
1850s; Délacour, 1966). Crow tool use is featured in New Caledonian
folklore and early European accounts, and stick tool use was reported by
Orenstein in 1972. However, it was not until 1996 that the complexity
and diversity of New Caledonian crow TOB was described by Gavin Hunt
(Hunt, 1996). While many species use tools (Beck, 1980), the habitual
use of multiple types of tool in the wild was thought to be restricted
to primates (e.g., Parker & Gibson, 1977). Hunt and colleagues have
demonstrated that, as a species, wild crows use a variety of tools,
which they classified into three categories: straight sticks or leaf
stems (Hunt, 2000b; Hunt & Gray, 2002; Rutledge & Hunt, 2004), hooked
twigs or vines (Hunt, 1996; Hunt & Gray, 2004a), and tools torn from the
leaves of the Pandanus tree (Figure 3; Hunt, 1996, 2000a; Hunt et al.,
2001; Hunt, Corballis, & Gray, 2006; Hunt & Gray, 2003, 2004b). Like
some other corvids (Cristol & Switzer, 1999), New Caledonian crows drop
nuts to break them open (Layard & Layard, 1882), but using an unusual
technique they wedge the nuts in tree forks before rolling them off
(Hunt, Sakuma, & Shibata, 2002), perhaps to increase accuracy or
efficacy of dropping.
The shape of tools the
crows produce from Pandanus
leaves varies across New Caledonia, leading to the suggestion that
tool-manufacturing skills may be transmitted and maintained culturally,
perhaps even with a human-like ratchet effect where innovations are
accumulated over time (Hunt, 2000a; Hunt & Gray, 2003). Since these data
are observational, rather than experimental, and are based on the
collection of tool counterparts made by an unknown number of
individuals, the intriguing possibility of cultural transmission remains
an untested hypothesis (see Laland & Janik, 2006). One of the main
difficulties in resolving this issue is that it is not yet possible to
compare variation in tool shapes produced by individuals from different
populations, which requires the observation of large samples of
individually-identifiable birds.
Very little is currently
known about the behavioural and social ecology of these crows in the
wild, other than that they are social, that juveniles remain in some
proximity to their parents for relatively long periods, and that they
are found in dry and humid forest habitats in New Caledonia and in
savannah and agricultural areas (reviewed in Kenward, Rutz, Weir,
Chappell, & Kacelnik, 2004). Field studies with marked individuals by
both research groups are underway (Figures 4 and 5), but until further
reports are available there is hardly any basis for speculation about
the reasons for their unique tool-related specialisation.
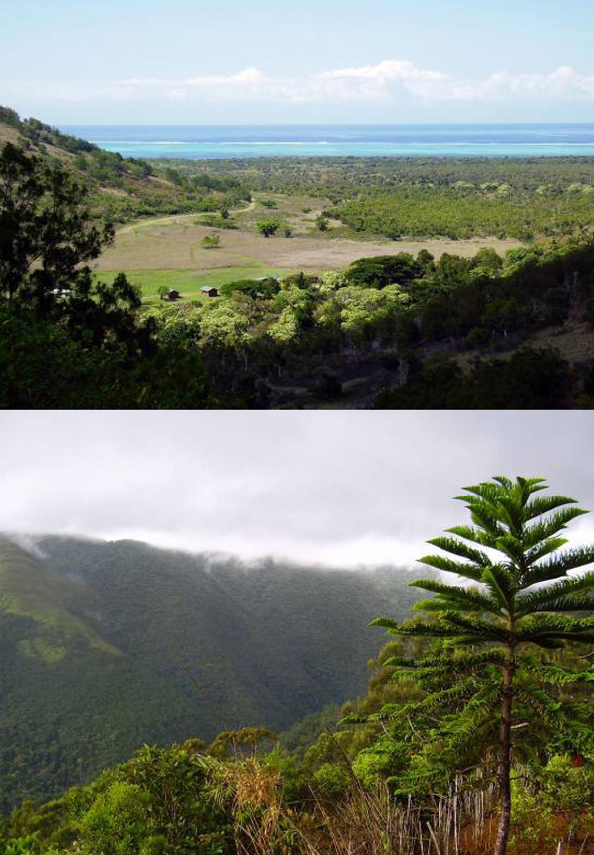
Figure 4. New Caledonian crows
are found across the main island in a range of habitat
types. The images show two of our present study sites in
dry (top; note base camp at centre of image) and humid
forest (bottom). Photos: Lucas Bluff and Christian Rutz
(reprinted with permission). |
|

Figure 5. Wood-boring beetle
larvae (Cerambycidae; top) live in dead Bancoulier
trees. New Caledonian crows use stick tools to probe
into their burrows and extract the larvae; sometimes
tools can be found still inserted into holes (bottom).
Photos: Lucas Bluff and Christian Rutz (reprinted with
permission). |
|
Development of Tool-oriented Behaviour
When observing fluent tool
use by an adult New Caledonian crow, it is hard even for seasoned
behavioural scientists to avoid interpreting the behaviour in terms of
planning and understanding. It is perhaps the presence of these first
impressions that highlights the importance of a program of research into
the evolutionary, ecological, ontogenetic, and cognitive underpinnings
of what the crows do. All classical ethological questions, from
causation and development to function and phylogenetic history, are
pertinent and hard to answer. Various hypotheses about causation and
concomitantly about the reasons why similar behaviour is not observed in
other avian species can be used to frame the problem. The following are
some possibilities: (a) TOB may result from a set of genetically
determined rules, in the sense applied to most animal architecture
(e.g., nests, spiders’ webs; Hansell, 1984, 2005); (b) TOB may develop
because of particularly advanced reasoning abilities, with individuals
‘working out’ how to solve problems using tools—an inherited capacity
for rational insight or a habitat offering special opportunities during
ontogeny would explain the rarity of the trait; (c) juveniles may have
an inherited tendency to manipulate physical objects at random,
gradually converging onto proficient tool use by their own history of
reinforcement—in this case, the predisposition for object manipulation
(and not for tool use) would be the relevant inherited trait
characterising the species; or (d) the behaviour may be passed on
through imitation of other tool-using individuals. Hypothesis (d)
ignores the problem of how TOB was acquired by the population in the
first place and implies that TOB may not be an adaptive specialisation
in itself, but a consequence of an enhanced general tendency for social
learning.
These hypotheses are not
mutually exclusive, and it is reasonable to expect that a grain of truth
may be associated with each of them and also with other possibilities.
One way to eliminate some, however, is to monitor the development of
naïve juveniles under controlled conditions. The degree of spontaneous
development of behaviour sets boundaries to the need to invoke learning
or social transmission and also serves to establish to what extent TOB
is an evolved adaptation in this species. Distinguishing between
reasoning and a tendency for object manipulation (hypotheses [b] versus
[c], respectively) is more difficult, but one might expect a gradual
emergence of tool use under hypothesis (c), with non-functional object
manipulation persisting after the emergence of successful tool use,
whereas reasoning should lead to a sudden acquisition of successful
behaviour, with a fast reduction in inefficient behaviours.
In the summer of 2004, we
reared four crows in our Oxford laboratory (Kenward, Rutz, Weir, &
Kacelnik, 2006; Kenward, Weir, Rutz, & Kacelnik, 2005). Three
individuals hatched from artificially-incubated eggs, and a fourth was
removed from the nest one day after hatching. We investigated the
development of two aspects of their TOB: stick tool use, and Pandanus
tool manufacture.
Development of Tool Use
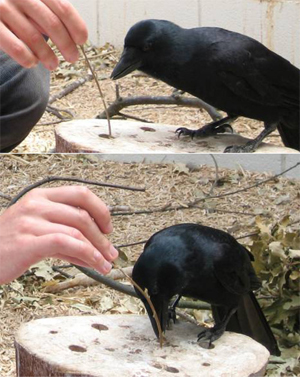
Figure 6. A captive-bred juvenile
crow watches a human tutor demonstrating tool use.
Photos: Charlotte Burn (reprinted with permission). |
|
To make the best use of our
limited sample size, we decided to expose different juveniles to
different rearing environments. From the time they started to venture
outside their artificial nests (a stage called ‘branching’), two of the
crows (‘Uek’ and ‘Nalik’) received daily demonstrations of tool use (but
not of tool making), in which a human foster parent extracted food from
holes and crevices (Figure 6). The other two juveniles (‘Corbeau’ and
‘Oiseau’) never saw tool use, but otherwise received the same degree of
overall contact with their human caretakers and, importantly, were fed
near holes to control for the possible effect of local enhancement. One
of these untutored birds (Corbeau) never saw any tool-like object being
handled, but the other subject (Oiseau) may have observed a few
accidental instances of a keeper picking-up sticks from the floor of the
aviary (although not using them in any purposeful way).
By the age of 79 days, all
four juveniles retrieved food from crevices with stick tools (Figure 7;
Movie 1), with no obvious difference in the onset of tool using between
individuals from different treatment groups (Kenward et al., 2005).
These observations are sufficient to discard extreme versions of
hypotheses (b) and (d) listed above: Although systematic comparisons are
still lacking, other corvids such as magpies (Pica pica), western
scrub-jays (Aphelocoma californica), European jays (Garrulus
glandarius), jackdaws (Corvus monedula), rooks (C.
frugilegus), common ravens (C. corax), and American-,
carrion-, and jungle crows (C. brachyrhynchos, C. corone, C.
macrorhynchos) are often hand-reared for both scientific research or
as pets, but none shows a tendency to develop tool use spontaneously.
Anecdotal episodes of tool use in wild and captive corvids have been
reported (Andersson, 1989; Boswall, 1978, 1983; Caffrey, 2000; Cole,
2004; Gayou, 1982; Jones & Kamil, 1973; Reid, 1982), but all of these
are examples of unusual, idiosyncratic behaviour in one or a few
individuals (for example, one American crow observed by Caffrey (2000)
broke off and used a tapered piece of wood as a tool, of a similar shape
to the stepped-cut Pandanus tools made by New Caledonian crows). The
time-course and nature of tool-use development was strikingly similar
between all of our subjects, including those that were completely
untutored. In this regard, the crows appear to be similar to other birds
that routinely use tools: Tool use develops spontaneously in woodpecker
finches (Cactospiza pallida; Tebbich, Taborsky, Fessl, &
Blomqvist, 2001), the only other bird species that habitually uses stick
tools in the wild; in Egyptian vultures (Neophron percnopterus;
Thouless, Fanshawe, & Bertram, 1989), which use stones to crack open
ostrich eggs; and in hyacinth macaws (Anodorhynchus hyacinthinus;
Borsari & Ottoni, 2005), which use slivers of wood or leaves as wedges
while cracking nuts.

Figure 7. Hand-reared juveniles
started probing into holes (top) and artificial crevices
(bottom) at approximately the same age, regardless of
whether they had been tutored or not. Top: from Figure 1
(a) (photo: Lucas Bluff), page 1331, "Development of
Tool Use in New Caledonian Crows: Inherited action
patterns and social influence," by B. Kenward, C. Rutz, A. A. S. Weir, and
A. Kacelnik, Animal Behaviour, 72, 1329-1343. Copyright
2006 by The Association for the Study of Animal
Behaviour. Adapted with permission. Bottom: from Figure
1a (photo: Ron Toft), page 121, "Tool Manufacture by
Naive Juvenile Crows," by B. Kenward, A. A. S. Weir, C.
Rutz, and A. Kacelnik, Nature, 433, 121. Copyright 2005
by Nature Publishing Group. Adapted with permission. |
|
Food retrieval in all
hand-reared New Caledonian crows was developmentally preceded by
stereotyped object-manipulation patterns (so-called ‘precursor
behaviours’) that contained components of the functional, mature
behaviour (Kenward et al., 2006). For example, all juveniles engaged in
‘proto-probing’ (first observed in the second week post-branching),
where the bird holds a twig in its beak and moves its head
back-and-forth in a manner that would be appropriate for probing a hole
or crevice to extract food; in this case, however, the twig is moved
whilst touching against the surface of another substrate (such as a
perch), rather than being inserted into a concavity. Precursor
behaviours have also been reported for the development of tool use in
the woodpecker finch (Tebbich et al., 2001), and for various other
object-oriented behaviours in a range of bird species, including
snail-smashing in song thrushes (Turdus philomelos; Henty, 1986),
caching in Parids (Clayton, 1992), and nest-building in village
weaverbirds (Textor cucullatus; Collias & Collias, 1964).
The presence of precursor
behaviours in all our hand-reared crows begs the question of whether
they play a role in enabling the developing crow to learn about the
consequences of object manipulations by honing the functional TOB that
develops at later stages. This kind of ‘perception-action’ development
is believed to be central to the development of tool-oriented behaviours
in the human child and other primates (Gibson & Pick, 2000; Lockman,
2000). The crows’ precursor behaviours did not result in (and hence were
not shaped by) reinforcement through food acquisition—all precursor
actions were performed weeks before successful food retrieval was
observed. This eliminates the possibility that tool use develops as the
result of an increased tendency for purely random object
manipulation (hypothesis[c]), and emphasises the role of inherited
traits together with some form of ‘internal reinforcement.’ Of course,
this finding does not reduce the significance of learning, which indeed
may itself be strongly based on specific predispositions related to tool
use (for further discussion, see Kenward et al., 2006). The development
of tool use without social input, the existence of inherited precursor
behaviours specific to tool use (namely stereotyped tool-related actions
that emerge without reinforcement), and the fact that New Caledonian
crows frequently use tools in the wild, indicates that tool use has been
specifically selected for and therefore that the behaviour is, or was in
the past, an adaptive specialisation.
In chimpanzees (Pan
troglodytes) and capuchins monkeys (Cebus apella), a long
period of object exploration and individual learning precedes functional
tool use, with a notable increase in behavioural complexity during
development (chimpanzees: Biro et al., 2003; capuchins: Fragaszy &
Adams-Curtis, 1997; Lonsdorf, 2005). Furthermore, these species seem to
have a predisposition for certain action patterns, such as insertion of
objects (capuchins Cebus apella: Fragaszy & Adams-Curtis, 1997;
chimpanzees Pan troglodytes: Hayashi & Matsuzawa, 2003). The
ontogeny of TOB in crows (Kenward et al., 2006) is similar to that of
capuchin monkeys (Fragaszy & Adams-Curtis, 1997) in that non-functional
(precursor) behaviours persist until after successful tool use has
developed. As a conceptual model, the ontogeny of TOB seems to follow a
similar path to the development of song in some passerines and language
in humans: Members of the species are endowed with a host of genetic
predispositions to acquire the behaviour, but learning—and in the case
of song and language, specifically social learning—determines the
fate of these predispositions (Marler & Slabbekoorn, 2004). It is thus
necessary to consider to what extent social factors can affect the
development of TOB in New Caledonian crows.
While the onset of tool use
did not differ between birds of the two treatment groups, other
object-related aspects of development did. Compared to the untutored
birds, the two tutored subjects spent more time performing activities
related to tool use, such as twig carrying and inserting, but did not
differ in measures of general motor development, such as locomotion and
carrying of non-food, non-twig items (Kenward et al., 2006). The
sensitivity of crows to social factors was further highlighted by the
results of a simple choice experiment (Kenward et al., 2006). Tutored
juveniles were allowed to observe a human manipulating an object (the
object was simply turned in the hand, not associated with any other
object) and then given a choice between this and a similar object that
had also been present but had not been manipulated by the tutor. Both
birds had a strong preference for manipulating the objects that had been
handled by the human experimenter. In the wild, such stimulus, local
enhancement, or both may play an important role in the acquisition of
certain aspects of TOB, such as the choice of appropriate raw materials
for tool manufacture. In primates, notably chimpanzees, there is no
doubt that social learning does play a significant role in the ontogeny
of object-oriented behaviours (e.g., Lonsdorf, 2005, 2006). On the other
hand, no effect of tutoring by conspecifics was detected on TOB
development in woodpecker finches (Tebbich et al., 2001).
Development of Pandanus Tool Manufacture
When crows tear a tool out
of a Pandanus leaf, a distinctive ‘counterpart’ is left behind
(Figures 2b and 3). According to island-wide surveys by Gavin Hunt and
colleagues, Pandanus
tools conform to three distinct types—narrow, wide, or stepped—and the
relative frequencies of these types vary across New Caledonia without
any obvious association with relevant environmental factors (Hunt,
Corballis, et al., 2006; Hunt & Gray, 2003). This observation
constitutes the basis for the suggestion of a cumulative form of
cultural transmission of tool technology. Our experimental results show
that New Caledonian crows pay attention to the behaviour of others,
which is a prerequisite of the culture hypothesis. However, other
important pieces of the puzzle are whether socially isolated juvenile
crows can successfully manufacture Pandanus tools, and if they
do, whether they can produce the different tool shapes observed in the
wild. The greater the degree of competence shown by untutored animals,
the less we need to invoke social determinants.
We first formally tested
the response of our hand-reared juveniles to Pandanus leaves when
they were between 3 and 4 months old (the two tutored birds were exposed
to Pandanus
around 2 months old, but were too young to tear at the leaves). To do
so, we mounted individual Pandanus leaves on frames in the
juveniles’ aviaries, and recorded their behaviour (no birds received
demonstrations). All four subjects immediately ripped shreds off the
leaves, and one bird (Corbeau) manufactured and used a functional tool
on his first day of exposure to the leaves (Movie 2; Kenward et al.,
2005). All subjects ripped off leaf fragments that could have been used
as tools, although only Corbeau was actually observed extracting food
with them.
In a subsequent experiment,
when the juveniles were between 6 and 7 months old, the two subjects
that previously received demonstrations of tool use (Uek and Nalik) were
now allowed to observe a human making tools from leaves and using these
to retrieve food (but not to keep them or use them themselves). One of
the untutored subjects (Corbeau) was given four human-made tools plus
intact Pandanus
leaves, and the final untutored subject (Oiseau) was exposed to intact
leaves but no tools. All subjects received three leaves (mounted more
naturalistically than previously, on an artificial ‘tree’) over 5 days,
apart from Oiseau, who only received two due to a shortage of available
leaves. Functional tools were produced by the tutored subjects (4 tools)
and the subject (Corbeau) who had been provided with pre-made tools (12
tools), but not by the subject (Oiseau) simply exposed to intact leaves;
all four juveniles continued to produce non-functional strips (Kenward,
2006). This is consistent with the result of the first experiment:
namely, that captive-born New Caledonian crows will tear tool-shaped
strips from Pandanus leaves in the absence of social inputs, and
hence without a requirement for culture. However, the pieces removed
from the leaves and used as tools were crude in shape and manufactured
using a different technique from that used by (at least) one wild crow
observed by Hunt and Gray (2004b). Therefore, the possibility remains
that social influence is important in promoting and refining Pandanus
tool manufacture in wild crows.
The failure to produce
refined stepped tools could have occurred for several reasons, some of
which are supportive of the culture hypothesis while others argue
against it. Reasons why invoking culture may not be necessary include
the following:
1. Environmental limitations. The lab experiments
may underestimate the tool-making ability of untutored birds.
Reasons for this may be that the leaves available to the crows in
Oxford were not from the same (sub)species of Pandanus that
is typically used by wild crows in New Caledonia, and differences in
leaf morphology may well have affected the juveniles’ performance.
Moreover, the leaves were presented on an artificial mount, rather
than as part of a natural tree, and the mode of accessing the leaf
might affect manufacture behaviour. Finally, the juveniles were
raised in confined areas and provided with food ad libitum,
so a lack of nutritional need and a surplus of ‘free time’ may have
reduced the incentive for them to refine tool manufacture.
2. Developmental limitations. The birds were
still young, and refined tool manufacture may require further
maturation of fine motor skills. Also, the juveniles may have been
given access to raw materials too late during their development, or
given insufficient exposure to leaves and opportunity to practice.
We only had a limited supply of fresh Pandanus leaves and
only offered them on a handful of occasions.
3. Individual differences. It is possible that
only some individuals are proficient Pandanus tool
manufacturers, and our small sample may not have included any such
birds (the level of individual specialisation in the wild is not yet
known).
4. Genetic differences. Pandanus-tool
manufacture may require specific genetic adaptations, and since (to
our knowledge) none of our wild-caught captive crows come from areas
of New Caledonia where Pandanus tools are made (see Kenward
et al., 2004, for details of capture locations), our captive-bred
juveniles may lack requisite genetic adaptations.
In support of the culture
hypothesis, it could be that, while some aspects of TOB are narrowly
canalised genetically, social learning is a prerequisite for the
acquisition of specific manufacturing techniques, tool designs, or both.
These options are clearly separable with further data, and forthcoming
research will clarify the issue.
Social learning need not
involve faithful imitation of the exact motor actions exhibited by
tool-producing conspecifics. More rudimentary social processes such as
stimulus or local enhancement (Galef, 1988; Heyes, 1994), as
demonstrated in our choice experiment mentioned above, could expose wild
juveniles to a biased set of raw materials and hidden prey. Juveniles
may also learn about particular
Pandanus tool shapes from inspecting abandoned tools or tool
counterparts in leaves, without ever observing another bird
manufacturing a tool. In other words, the hypothesis of a tool culture
is compatible with various social transmission mechanisms, ranging from
elaborate cognitive properties to better-known and widespread learning
processes.
It is interesting to view
our experimental findings in the light of first results on juvenile
development in the wild. We have monitored radio-tagged wild crows from
2005 onwards, and our preliminary data suggest that juveniles stay in
(extended) family groups for at least the first year of life, foraging
often in close association with parents, siblings, and other
individuals. This period of dependency prior to natal dispersal could
provide opportunities for social transmission of information: vertically
(from parents to offspring), obliquely (from the parental to other
members of the offspring generation), or horizontally (between juveniles
of different ages). There are also very suggestive observations of
juveniles watching their parents using tools and subsequently using the
same tools (Hunt, 2000b; Sarsby, 1998).
In conclusion, proficient
TOB in New Caledonian crows results from a complex interplay of
heritable predispositions, individual learning through object
exploration, and (quite possibly) the acquisition of
socially-transmitted information. In conjunction with further work on
captive subjects, the long-term observation of individually identifiable
wild subjects will be instrumental for teasing apart the relative
contributions of these different mechanisms.
Deployment of
Tool-oriented Behaviour
The fact that at least some
aspects of New Caledonian crows’ tool-oriented behaviour develop from
unlearned, heritable predispositions does not imply that TOB must be
stereotyped or unrelated to reasoning in adult crows. Consider again our
earlier analogy with the development of human language. A prevalent view
argues that there is an inherited basis for the mechanisms of human
language acquisition (Vargha-Khadem, Gadian, Copp, & Mishkin, 2005; but
see Fitch, Hauser, & Chomsky, 2005), but it would be unreasonable to
maintain that cognition is absent from conversation. Tools are an
unusual way of interacting with the physical world. Therefore,
independent investigation is necessary to explore the cognitive
underpinnings of TOB deployment by adult crows—their folk physics, or
"common sense understanding of how the world works and why it works in
the way it does" (Povinelli, 2000, p. 9). It is worth noting that, while
tool use may be favoured by the pre-existence of a high level of
understanding (as some argue for humans; e.g., Wolpert, 2003), the same
behaviour, whatever its control mechanism, may promote the evolutionary
development of folk physics by exposing the subjects to situations where
understanding the causal basis of interactions between objects gives
them a competitive advantage, thus reversing the arrow of causality.
Another possibility is that exposure to extensive object-object
interactions during a juvenile period promotes the development of folk
physics and that this tendency to play with objects is the
species-specific cognitive adaptation responsible for the unique level
of tool use.
In the next section, we
first examine how selective and flexible adult crows are in making and
using tools, providing hitherto unpublished re-analyses of data, and
then we consider whether crows may possess anything worthy of the term
‘understanding.’
Choosing Tools
A fundamental feature of
intelligence is flexibility (Emery & Clayton, 2004b; Lefebvre, Reader, &
Sol, 2004; Reader, Sol, & Lefebvre, 2005; Roth & Dicke, 2005): being
able to alter one’s behaviour if circumstances change (John Maynard
Keynes famously replied to a fellow parliamentarian who accused him of
inconsistency that "When the facts change, I change my mind. What do you
do, sir?"). Particularly diagnostic of intelligence is the ability to
alter one’s behaviour
adaptively: that is, to assess how to change one’s behaviour to cope
best with the change in circumstances. Therefore, perhaps the first
question to ask of a tool user is whether s/he can choose the best tool
out of a selection of tools of different dimensions when the task is
varied. If tool use is based on rigid rules for choosing particular
tools in particular situations, then we would expect that, when
confronted with novel problems (i.e., those not typically encountered in
natural contexts), subjects would continue with their default behaviour
or choose at random. Choice of the correct tools in novel tasks would at
least indicate that the natural behaviour was not rigidly specified. It
is less clear whether such flexibility indicates the involvement of
reasoning or abstract concepts, although, depending on the task, choice
could be governed by previously learned associations between particular
tools and success or by an understanding of the relationship between
tool dimensions and task demands.
Wild chimpanzees use tools
of different dimensions for different tasks (e.g., Boesch & Boesch,
1990, 1993; Hicks, Fouts, & Fouts, 2005; Nishida, 1972). This has been
taken as evidence for selectivity and ‘mental representation’ of task
demands (Boesch & Boesch, 1993; Visalberghi, 1993). However, since the
chimpanzees were not described as solving novel problems, it is
difficult to assess the extent of individual flexibility. In controlled
experiments, captive gorillas (Gorilla gorilla) and orangutans (Pongo
pygmaeus) appropriately chose the longer of two tools to rake in a
food reward when it was distant and chose randomly between the tools
when the reward was close (Mulcahy, Call, & Dunbar, 2005; see also
Pouydebat, Berge, Gorce, & Coppens, 2005), as did a Tonkean macaque (Macaca
tonkeana) in a similar task (Ueno & Fujita, 1998). Two capuchins
also spontaneously chose the thinner of two sticks when necessary
(Anderson & Henneman, 1994). In contrast, woodpecker finches did not
spontaneously choose longer tools when food was further away (Tebbich &
Bshary, 2004). In Tebbich and Bshary’s experiments, five birds were
given sticks of five different lengths and food at five different
distances inside a horizontal transparent tube. On their first trials,
all subjects chose short tools, and across all trials the length of the
tools they first used did not correlate with the distance to food,
although over the course of the experiment three subjects did appear to
choose sufficiently long tools more frequently than expected by chance
(Tebbich & Bshary, 2004).

Figure 8. The shortest tool
chosen at each food distance by two subjects (‘Abel’:
black circles, solid line; ‘Betty’: red squares, dashed
line) in a length selection experiment. Trend lines are
linear regressions, and the data for Abel are pooled
over the two experiments he participated in. In the
original analysis, two kinds of choices were shown to be
significantly above random: those of the tool whose
length matched the distance to food and those of the
longest tool. As the bias towards choosing the longest
available tool might obscure a sensitivity to distance
to food, here we exclude all such choices (15 trials for
Abel and 10 for Betty). Data are replotted from Figures
2 and 4, pages 74 and 76, "Tool Selectivity in a
Non-mammal, the New Caledonian Crow (Corvus moneduloides),"
by J. Chappell and A. Kacelnik, Animal Cognition, 5,
71-78. Copyright 2002 by Springer-Verlag. Adapted with
permission. |
|
A relevant question is,
therefore, whether New Caledonian crows can choose tools of appropriate
dimensions for novel problems. This was first assessed by giving two
subjects (‘Betty’ and ‘Abel’) tools of ten different lengths and food at
different depths inside a transparent, horizontal tube (Chappell &
Kacelnik, 2002). Both subjects selected appropriate tools from their
first trials and chose tools that were too short to reach food on just
13% of trials, against a chance expectation of 45%. Each subject chose
the tool that exactly matched the distance to food on 5 of their first
20 trials and the longest available tool on 10 trials (both
significantly greater than expected by chance). In addition, new
analysis of the data reveals that, excluding all trials in which the
longest tool was chosen, there is a significant increase in minimum tool
length chosen by each subject at each distance to food (Figure 8;
Spearman rank correlation; for Betty [who participated in only one
experiment], N = 7, rS
= 0.764, p < 0.05; for Abel [who participated in two
experiments], on pooled results from both experiments N = 10,
rS = 0.652, p < 0.05]).
The subjects were also
tested on a task where they had to select tools of the appropriate
width. Here, a tool could be inserted through a hole of three possible
diameters to dislodge a food reward (Chappell & Kacelnik, 2004). In the
first experiment, Betty was given three tools of different diameters (1
mm less than the diameter of the three holes) in different
configurations: either all loosely tied into one bundle, or two tied
together and one free. Betty showed a strong preference for the tool
with the smallest diameter, to the point of dismantling the bundle to
retrieve it even when a suitable tool was freely available. That she
would incur this additional handling cost suggests selectivity (she
strongly preferred one tool) at the expense of flexibility (other tools
were suitable, but she did not use them). In a control condition, where
all the tools were available and suitable for food-retrieval, Betty
showed the same preference, suggesting that her affinity for the
narrowest tool may have been driven by ergonomic factors.
 |
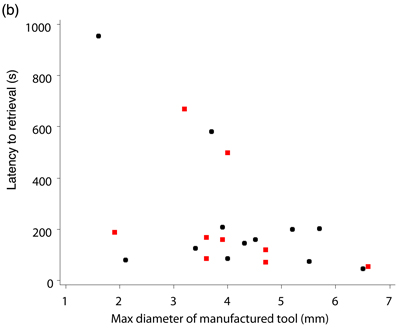
Figure 9. (a) Maximum diameter of
the functional region (distal 9 cm) of the manufactured
tool plotted against the diameter of the hole, in a
diameter-manufacture experiment. Horizontal jitter has
been added to separate overlapping data points. The blue
line represents a linear regression through all
unmodified tools. Data are replotted from Figure 4, page
125, "Selection of Tool Diameter by New Caledonian Crows
Corvus moneduloides," by J. Chappell and A. Kacelnik,
Animal Cognition, 7, 121-127. Copyright 2004 by
Springer-Verlag. Adapted with permission. (b) Latency to
food retrieval plotted against the maximum diameter of
the functional region of the manufactured tool for two
subjects (Abel: black circles; Betty: red squares). Only
trials where just one tool was manufactured and used
(unmodified) successfully are included. Note that the
statistical analysis was performed on transformed
latencies (see text for details), whereas the
untransformed data are plotted here for ease of
interpretation. Unpublished data from Experiment 2,
"Selection of Tool Diameter by New Caledonian Crows
Corvus moneduloides," by J. Chappell and A. Kacelnik,
Animal Cognition, 7, 121-127. |
|
The previous test was
concerned with tool use, but we also conducted experiments to
investigate aspects of our crows’ tool manufacture. Both Betty and Abel
were tested together on the same apparatus as used for the
width-selectivity test, but with access to raw materials for tool
manufacture (an oak branch;
Movie 3) rather than artificial tools.
In each trial, the subjects approached the tube and looked at it before
making tools. Out of 30 trials, the male (Abel) retrieved food on 13 and
the female (Betty) on 16 (the remaining trial was aborted because
neither subject had obtained the food after 30 minutes). Abel retrieved
food using the first tool he made (without modifying it) in 13 trials,
only making an inappropriate tool on 1 trial (the tool was narrow
enough, but too short to dislodge the food). Betty retrieved food using
the first (unmodified) tool she made on 10 trials, and on 2 trials she
modified the tool to make it narrower after trying to use it (Movie 4).
On 5 trials, her first tool was too short, although suitably narrow, and
on 4 of these she made another tool that she used to retrieve food.
Pooling results from both subjects, the maximum diameter of the
(unmodified) tools increased significantly with the diameter of the hole
(Figure 9a; Chappell & Kacelnik, 2004). Strikingly, 16 of the 24 tools
made in middle- and large-hole trials would have been too thick if used
on small-hole trials, whereas only one tool made in the narrow-hole
trials was too thick (and it was subsequently modified to make it
narrower). There is no quantitative information on how the crows may
benefit from selectivity in this task (as opposed to making exclusively
narrow tools that are functional for all three hole diameters), but a
post hoc analysis of one aspect of the results not examined in the
original publication reveals a potential and perhaps non-obvious
association between tool manufacture and foraging efficiency. Whilst the
latency to success (from the start of the trial) showed no trend with
hole diameter, this latency decreased with diameter of the manufactured
tool (only including trials where the first tool made was successfully
used, unmodified; Figure 9b; GLM analysis with ‘latency to success’
[transformed by reciprocal square root] as the dependent variable, tool
diameter as a continuous explanatory variable, and subject as a random
explanatory variable; for tool diameter, F1,18
= 7.95, p = 0.011; for subject, F1,18
= 0.04, p = 0.848, F1,24
= 0.18, p = 0.671]. This is consistent with the hypothesis that
the subjects were being selective only when required since the narrower
the desired tool diameter, the fewer the tools that fulfil the criterion
and the longer the requisite search time until a suitable twig is found.
Thus, crows seem capable of manufacturing appropriately-sized tools from
raw materials as well as of choosing from a set of available tools
according to the present needs, although their choice may also be
constrained by inherent preferences, whether these are the product of
previous learning or simply ergonomic limits.
A somewhat different
picture of flexibility emerges from field experiments. Hunt, Rutledge,
and Gray (2006) examined how two free-living New Caledonian crows made
(or chose) and used tools to extract food from an artificial
container—either a vertical box with a transparent side, or opaque
vertical holes in a tree stump. Food was placed at one of two depths,
which varied between blocks of trials. One subject was given
Cerambycidae larvae (Agrianome fairmairei, provided alive or
dead; see Figure 5, top panel) and used mainly leaf stems to extract
them, while the other manufactured Pandanus
tools to extract supplied lumps of meat. The main finding was that the
depth of the hole did not affect the length of the first tool with which
either crow attempted to extract food (this tool tended to be shorter
than the distance to food in the deeper holes), and only after failure
to retrieve food with the first tools did the subjects make or select
longer tools. Therefore, there was no evidence that the subjects
assessed the distance to the food before choosing tools, although they
did adapt their behaviour once the tools had been tried. The authors
argue that this demonstrated that the crows "may generally use a
two-stage heuristic strategy to solve tool problems" (Hunt, Rutledge, et
al., 2006, p.307)—namely, initially using default behaviour and
modifying this appropriately following failure. They argue that this
rules out the possibility that the crows were using ‘immediate causal
inference’ to solve the problem and that the adaptive modification of
tool length following failure was consistent with either a ‘previously
developed associative learning rule’ or ‘delayed causal inference’
(Hunt, Rutledge, et al., 2006).
These observations contrast
with those of Chappell and Kacelnik (2002, 2004) described above, who
found selectivity and flexibility for length and diameter from first
attempts in a new task. In our opinion, however, major methodological
differences between the studies preclude direct comparisons. Of
particular relevance is the fact that the study by Hunt, Rutledge, et
al. (2006) used free-living crows and a naturalistic foraging task.
Consequently, experimental trials were embedded within a natural
foraging context, where the crows (presumably) routinely used tools.
Free-living birds are likely to develop a preference for using a tool
length that is sufficient to reach prey in most natural holes, a view
that is supported by our own ongoing work on stick-tool use in wild
crows. If this is the case, crows may have approached the task in Hunt,
Rutledge, et al.’s experiment with their default behaviour, not noticing
that it required a different kind of tool (the ‘two-stage heuristic
strategy,’ in Hunt, Rutledge, et al.’s terminology). In contrast, in
Chappell and Kacelnik’s laboratory studies, experimental trials
represented the majority of the daily tool-related foraging by the
subjects, and the novel nature of the task (the distance to food varied
between consecutive trials, rather than between blocks of trials, and
food was presented in a highly distinct, novel apparatus) may have
elicited case-by-case decision making. The nature of the apparatus
(vertical opaque- or partially-opaque-sided holes for Hunt, Rutledge, et
al.’s subjects; a horizontal transparent tube for the Oxford subjects)
also provides a possible explanation for the different results. The
subjects in Hunt, Rutledge, et al.’s experiments may not have exposed
their ability to discriminate before choosing the initial tools because
they simply were not able to assess the distance to food in vertical,
opaque, or semi-opaque holes. Other methodological differences hamper
comparisons. For instance, Hunt, Rutledge, et al.’s study used two food
depths in separate blocks of trials, whereas Chappell and Kacelnik’s
(2002) experiment exposed subjects to 10 distances to food in randomly
interspersed trials. These disparities highlight the complementarity of
field and laboratory studies. While the former are irreplaceable for
investigating ecological relevance, the latter allow for stricter
control of the subjects’ state and experience and demonstrate abilities
that may or may not be important under natural circumstances. No doubt,
both approaches are necessary to elucidate detailed mechanisms of
learning and choice.
In conclusion, New
Caledonian crows, like some tool-using primates, are able to select
appropriate tools for novel tasks. In the laboratory, they make and use
tools from a variety of materials, including (their own moulted)
feathers, cardboard, and wood chips, and they readily use any elongated
object for probing holes and crevices, e.g., screws, strips of plastic,
or wire (Kacelnik, Chappell, Weir, & Kenward, 2006; personal
observations). Appropriate selection of tools based on length has not as
yet been demonstrated for wild crows (Hunt, Rutledge, et al., 2006), or
in a similar experiment in captivity with the other avian stick-tool
user—the woodpecker finch (Tebbich & Bshary, 2004). While selectivity
and flexibility are to be expected if behaviour is controlled by logical
inference and planning, their presence does not prove causal
understanding. For example, subjects could have a rich repertoire of
previous associations between tools of particular dimensions and holes
of a certain depth or diameter, which would enable them to choose
correctly in ‘novel’ tasks. Selectivity—defined as the use or
manufacturing of tools conditional in size or shape on the task being
faced—raises the question of which cognitive mechanisms are employed in
TOB, but it is not, per se, sufficient to identify any particular
ability that excludes conventional processes such as associative
learning plus generalisation.
Understanding?
As mentioned in the
introduction, humans possess intuitive abstract ‘theories’ about the way
the physical world operates, incorporating a variety of principles (such
as gravity, force, connectivity, weight) that allow us to operate as
obligate tool users in a materially complex environment and to predict
how objects will interact in novel situations. While this can be
described as causal understanding of the physical world, it need not
equate to formal understanding in any rigorous scientific or
philosophical sense. For example, by 12 years of age, children (who
presumably have experience using levers in their everyday life) are very
proficient when asked to judge how the position of weights along a scale
will affect the balance of a lever (for many, a novel task) (Amsel,
Goodman, Savoie, & Clark, 1996), yet they lack a formal understanding of
the underlying principles (that the mass multiplied by the distance must
be equal on both sides of the fulcrum) until this is explicitly taught
(Stephen Barlow, personal communication). Likewise, through experience
most people have an implicit knowledge of what makes an effective
hammer. If one forgets to bring a hammer on a camping trip, one can
choose another suitable object with which to knock in the tent pegs
regardless of superficial visual dissimilarities. This same rationale—a
task requiring transfer of concepts derived from past experience to a
novel problem—can be used to attempt to assess causal understanding in
non-humans.
To test for causal
understanding, two main experimental approaches have been used to date.
The first is another kind of selectivity test: Subjects are offered
choices either between different tools (where effective tools share a
common mechanism—such as ‘hooking’—but are perceptually different), or
between different actions with a tool (such as pushing or pulling a
tool, or choosing which side of an apparatus to insert a tool into).
Subjects are usually trained on a particular configuration of the
apparatus and are then tested with the task or tools transformed in a
manner which alters the causality of the problem. Transformations are
typically designed such that subjects who have learned ‘procedural
rules’ (sensu Povinelli, 2000) based upon superficial
characteristics of the apparatus (such as colour, shape, texture) will
behave in one way, whereas those that have formed a concept about how
the apparatus functions (for example, that the tool needs to be
connected to the food in order to act on it) will behave in another
way. However, since the number of options the subjects face is normally
limited, there is a relatively high chance that they will perform
correctly by chance. Therefore, a robust study requires either a large
sample of subjects to be tested, or a suite of different tasks or
transformations to be used. Furthermore, the limited number of options
and trials make one-trial learning very plausible, so relatively little
can be inferred from post-first-trial performance. For example, in a
task with only two options a win-stay lose-shift strategy would lead to
perfect performance after the first trial.
The second approach focuses
on the animals’ spontaneous behaviour. Rather than giving subjects a
defined set of choices, they are placed in a situation where they have a
low probability of solving a task by chance alone (for example, in a
hook making task an animal may be given a piece of pliable material that
can be changed into an infinite number of shapes, but only a small
subset of these shapes would be functional). Subjects with no causal
understanding might be expected to depend on trial-and-error and,
therefore, to follow a gradual pattern of acquisition similar to that in
classical operant shaping experiments (e.g., Thorndike, 1898). The
absence of insight should lead to gradual performance improvement
because success is achieved following a sequence of behaviours, any one
of which (from the subject’s perspective) could be the critical element.
In contrast, subjects that acquire and apply general principles might
equally rely on random exploration until success but then infer the
reasons for success and organise their future behaviour towards this
goal, hence improving in a more step-wise pattern. Experiments with
richer tasks, however, suffer from difficulties in formulating null
hypotheses and their respective probabilities (for example, how can one
define the likelihood of manufacturing a hook-shaped instrument to
extract a reward by chance alone?), and in comparing performances across
species. Animals differ in their behavioural repertoires and, therefore,
in their likelihood of solving given experimental tasks (cows, for
example, seem unlikely candidates for bending wire into hooks, despite
reports to the contrary by a British newspaper; Townsend, 2005).
Both approaches, therefore,
have their advantages and should be seen as complementary, but the
limitations of each of them should be taken into account when
interpreting results. We will return to the issue of what conclusions
can be drawn from such work in Section 6. We now turn our attention to
the performance of New Caledonian crows and other animals in the well
known ‘trap-tube’ test and discuss what can be deduced from these
results regarding the presence of physical understanding.
The Trap-tube Test
The most widely applied
test for causal understanding in the physical domain is the trap-tube
task (Visalberghi & Limongelli, 1994). In its simplest form, it consists
of a transparent horizontal tube with a recess (‘trap’) in the middle.
Food is placed in the tube and subjects are given a stick with which to
retrieve it. In the ‘active’ state the trap is oriented downwards; if
the subject moves the food across the central part of the tube, the food
falls into the hole and becomes trapped. In the ‘inactive’ state, the
trap is oriented upwards; here the subject can move the food either way
with impunity. Logically, a subject who understands gravity should never
move the food across the central part of an active tube, but should show
no such bias when faced with an inactive tube. In some experiments
another transfer test has been used, where post-training the trap is
positioned off-centre to test for use of a distance-based associative
rule (e.g., Limongelli, Boysen, & Visalberghi, 1995).
The only New Caledonian
crow tested to date (Betty) did not instantly solve the task, but
instead she improved gradually. After around 60 trials, she
spontaneously developed a two-stage technique. This involved first
inserting the stick in the tube from the ‘safe’ side (leaving the trap
between herself and the food) until its distal end protruded from the
opposite side, then walking to the opposite side and pulling the stick
so as to extract the food in a controlled manner. This technique avoided
the trap and had the added benefit of eliminating the danger of another
crow snatching the food, an event that could occur when pushing the food
out of the opposite side of the tube. Using a combination of this
technique and a standard pulling action (pulling the food towards her
from the side closest to the food), she reached criterion (obtaining the
food on 8 or more trials in three consecutive sessions of 10 trials)
after 110 trials (Movie 5; Chappell & Kacelnik, 2007; Kacelnik et al.,
2006). This time to acquisition is comparable to that of chimpanzees
(Limongelli et al., 1995; Reaux & Povinelli, 2000), capuchins
(Visalberghi & Limongelli, 1994) and woodpecker finches (Tebbich &
Bshary, 2004) tested on the same task, but comparability is difficult
because of Betty’s use of the two-stage technique. When the trap was
switched to the inactive state, Betty continued to avoid it using her
technique, even though it was now irrelevant. Chimpanzees (Reaux &
Povinelli, 2000) and capuchins (Visalberghi & Limongelli, 1994) also
continue to avoid the inactive trap, and consequently it has been argued
that they do not understand the causal basis of the task. In contrast,
one woodpecker finch reverted to chance behaviour in the transfer test
(Tebbich, Taborsky, Fessl, Dvorak, & Winkler, 2004). Two chimpanzees and
a capuchin monkey have been tested with the off-centre trap design,
following successful performance with the centred trap: The capuchin now
performed significantly below chance, demonstrating that she was
probably using a distance-based associative rule, whereas the two
chimpanzees remained highly successful (Limongelli et al., 1995).
Interesting as it is to
compare performance levels across species, the conclusions that can be
drawn from this general experimental design are limited (Chappell,
2006). One caveat is that it is not clear what the cognitive
implications are of failure to revert to random insertion after the trap
is inverted (Machado & Silva, 2003). Although logically it is not
necessary for the subject to carry on avoiding the ineffective trap, if
there is no cost to doing so (as in most published experiments) even
subjects who are capable of understanding might find no incentive to
modify their behaviour. Interestingly, even humans continue to avoid the
inverted trap, despite their understanding of the physical principles
involved (Silva, Page, & Silva, 2005). It is equally hard to draw
conclusions from observations where subjects do revert to random
tool insertion with the inverted trap. It is possible that such subjects
had learned through reinforcement the characteristics of the stimulus
(i.e., the tube with downward-oriented trap) so specifically that they
fail to generalise when the trap is inverted, and they react as if in a
completely new task, to which they therefore respond randomly.
Alternatively, subjects might monitor the moment-to-moment movement of
the food with respect to the trap; if the trap is inverted, the food
will never approach it closely, and subjects will therefore insert tools
randomly. This is thought to account for the performance of the one
woodpecker finch that ‘passed’ the transfer test (Tebbich et al., 2004).
There are similar problems with the off-centre trap test. Although
below-chance performance indicates that subjects were using a
distance-based associative rule, above-chance performance does not mean
that they understood the causal basis of the task. They might have been
using another associatively-learned rule, such as ‘push the food away
from the trap,’ without any understanding as to why they should do this.
To solve problems
associated with the standard task, Nicky Clayton and colleagues (Seed,
Tebbich, Emery, & Clayton, 2006; Tebbich, Seed, Emery, & Clayton, in
press) developed an alternative task to test the same principle, but
which could also be used by non-tool-using species—in their experiments,
rooks. To adapt the task to non-tool-users, wooden dowels with two solid
transparent disks attached (with the food reward positioned between
them) were pre-inserted into tubes, so subjects simply had to pull the
dowel to retrieve the reward. Various configurations of tubes were then
used to test the subjects.
In the study by Tebbich et
al. (in press), following training to pull the dowel out of a plain,
horizontal tube to get food, eight subjects were tested with a tube with
a single, off-centre trap. Those subjects that reached the criterion for
success on this task were then tested with a tube where food was placed
between two apparent ‘traps,’ only one of which was functional at any
time. This tube had two possible configurations (see Figure 10): In one
(Tube A), a black disc blocked one trap at the bottom and the other at
the top, so the subject had to move the food over the latter trap and
out the mouth of the tube; in the other (Tube B), one trap was again
blocked at the bottom by a black disc, but the second trap was open, so
food could be retrieved by pulling the dowel towards the trap with no
base, upon which the food would fall clear of the apparatus. In both
tube types, therefore, the active trap featured a black disc at its
base. Half the subjects were tested with Tube A first, and half with
Tube B, receiving between 40 and 50 trials with each. Three of the
subjects reached criterion performance (after 30, 40, and 50 trials) on
the single-trap tube, but all remained at chance throughout testing with
both two-trap tubes.

Figure 10. Modified trap-tubes
used for testing causal understanding in rooks. Tubes
A-B were used in both Tebbich et al. (2007) and Seed et
al. (2006), whereas tubes C-D were only used by Seed et
al. (2006). The arrow shows the path the food will take
if the stick is pulled in the correct direction. For
further explanation, see text. From Figure 2, page 699,
"Investigating Physical Cognition in Rooks, Corvus
frugilegus," by A. M. Seed, S. Tebbich, N. J. Emery, and
N. S. Clayton, Current Biology, 16, 697-701. Copyright
2006 by Elsevier Ltd. Adapted with permission. |
|
Seed et al. (2006) tested a
separate group of eight rooks in a very similar manner. Crucially
however, they did not first train the subjects on the single-trap tube.
Instead, half the subjects were trained with Tube A, and half with Tube
B. The seven subjects that reached criterion were tested on the
alternative configuration (i.e., those that received Tube A were tested
on Tube B, and vice versa). All 7 rooks were successful in extracting
food in their first trial with the new configuration, and they were
successful more often than expected by chance over 20 trials. These
results are consistent with the subjects learning the causal features of
the task but could also be explained by learning to avoid traps with
black discs at the bottom. To exclude the latter possibility, further
transfer tests were administered (see Figure 10). In Tube C, one trap
was blocked at the top and the other one had no base, and both ends of
the tube were blocked by bungs; food could be extracted through the
baseless trap, but subjects could only pull the stick once, as after the
first pull the distal end of the stick disappeared into the bung and
could not be pulled out again. Tube D had the same traps but no bungs in
the ends of the tube; however, the apparatus was lowered to the ground
such that food could only be extracted by pulling over the trap with the
blocked top. These tubes were designed so that the stimuli that
previously signalled which direction to pull the stick (such as "pull
away from a black disc at the bottom of trap") were changed or removed,
so that subjects responding on the basis of a single
associatively-learned cue would perform at or below chance. Subjects
were exposed to two blocks of ten trials of Tube C, followed by two
blocks of ten trials for Tube D, or vice versa. Six subjects were at
chance on Tubes C and D, but one rook, ‘Guillem’, showed near-perfect
performance on both from his first trial.
While appropriately
cautious of conclusions based on a single subject, Seed et al. (2006)
propose that Guillem possessed either an understanding of the observable
forces involved, or had abstracted the rules of the task (see also
Chappell, 2006). However, success on any one task, particularly one
involving a choice between just two defined actions, could be because
the subject happened, by chance, to learn about cues that were
causally-relevant for that task. For example, Guillem could have learned
that two cues predicted success: When the mouth of the tube is open,
pull the food towards the trap with the black disc on the top; and pull
the food towards a trap that has no black disc, if there is space
underneath the tube. The striking individual differences in performance
(only 3 of 7 rooks learned to solve the original trap-tube task in
Tebbich et al., in press, and none passed the transfer tasks; Guillem
was the only one of 8 subjects to pass the transfer tasks in Seed et
al., 2006) make this possibility more plausible, since if rooks were
predisposed to form theories about physical causality, we would expect
more of them to demonstrate this ability in the experiments. It is worth
comparing this work with that on capuchin monkeys.
The performance of capuchin
monkeys seems to be based almost entirely on trial-and-error learning of
procedural rules (Visalberghi & Limongelli, 1994; Visalberghi & Trinca,
1989). In some experiments, chimpanzees have shown greater sensitivity
to the causally-relevant aspects of the tasks (e.g., Limongelli et al.,
1995; Visalberghi, Fragaszy, & Savage-Rumbaugh, 1995), but these
successful performances fall short of demonstrating an understanding of
the physical mechanisms involved (see Povinelli, 2000). Sensitivity to
functionally-relevant features of tools has also been demonstrated by a
series of experiments by Marc Hauser and colleagues, primarily with
non-tool-using cotton-top tamarins (Saguinus oedipus; Hauser,
1997; Hauser, Kralik, & Botto-Mahan, 1999; Hauser, Pearson, & Seelig,
2002; Hauser, Santos, Spaepen, & Pearson, 2002; Santos, Rosati, Sproul,
Spaulding, & Hauser, 2005), but also by testing rhesus macaques (Macaca
mulatta; Santos, Miller, & Hauser, 2003), common marmosets (Callithrix
jacchus; Spaulding & Hauser, 2005), and vervet monkeys (Cercopithecus
aethiops; Santos, Pearson, Spaepen, Tsao, & Hauser, 2006). However,
these results suggest that the subjects possess domain-specific biases
regarding what features of objects are relevant in certain situations,
rather than an understanding of underlying causality (Penn & Povinelli,
2007). Indeed, in recent experiments Santos and colleagues have tested
tamarins on experiments similar to those used by Povinelli and
colleagues with chimpanzees (Povinelli, 2000) and apparently found that
like chimpanzees, the tamarins failed to distinguish between the
functional and non-functional tools or actions (reviewed by Hauser &
Santos, in press).
In conclusion, the result
from these experiments, and others employing a similar strategy of
offering subjects choices between tools or discrete actions (e.g.,
Povinelli, 2000, chap. 4-10; Visalberghi & Trinca, 1989), have yielded,
at best, very limited evidence for understanding of physical causality
in non-humans.
Hook Making
As discussed above, another
technique for investigating non-humans’ understanding of physical
causality is to observe their problem-solving behaviour in situations
that are too rich to be fully defined by a choice. To this end, we have
examined how New Caledonian crows manipulate pliant material to form
functional tools.

Figure 11. A crow in the Oxford
laboratory (Betty) uses a hook—which she has just made
by bending a piece of wire—to extract a bucket with food
from the bottom of a well (Weir et al., 2002). The
images do not come from the same trial. Photos: Alex
Weir (reprinted with permission). |
|
This line originates from
an unplanned observation in an experiment where Betty and another crow,
Abel, faced a choice between a straight or a hooked piece of garden
wire, with food available in a small bucket at the bottom of a vertical
transparent tube (Weir, 2006). The goal was to extend the tests of
flexibility and selectivity (see Weir, 2006, Section 4.1) to include
tool shape in addition to length and diameter. The apparatus was
designed such that the hooked wire was functional for retrieval of the
bucket, but the straight wire was not (this last restriction proved to
be almost, but not absolutely effective). On the fifth trial of this
experiment, Abel probed into the tube with the hooked wire but then flew
off with it to another part of the aviary before retrieving the bucket.
Betty attempted to lift the bucket with the straight wire, and when this
proved ineffective, proceeded to bend it into a hook, which she used to
extract the bucket (Figure 11; Weir, Chappell, & Kacelnik, 2002).
Although this has become a textbook example of animal intelligence
(e.g., Barnard, 2004; Boyd & Silk, 2006; Freeman, 2002) and was termed
"the most impressive example of folk physics in any animal" in a recent
review of bird cognition (Emery, 2006, p. 32; note that Emery wrote this
before the publication of the results of Seed et al., 2006), unravelling
the cognitive processes behind it presents serious challenges.
Perhaps the crucial issue
is whether Betty’s wire-bending demonstrates causal understanding. In 10
trials following the initial observation, Betty was only given a
straight wire and nearly always briefly attempted to retrieve the bucket
with the unmodified tool (Movie 6), but then she consistently bent the
distal end of it (i.e., the end not held in her beak) using two
different techniques both involving fixing the tip of the wire and
pulling laterally from the proximal end (Weir et al., 2002). The bent
part at the distal end formed the hook. (Note that we are using the term
‘hook’ informally to describe a bent piece of metal that could function
to pull the bucket out of the well; we recognise that, as pointed out by
Emery, 2006, in several cases the angle of the bend was less than 90
degrees.) Betty had been wild-caught as an immature bird two years
before the experiment took place, so her experience before entering
captivity is unknown, but we are not aware of any natural materials that
could be bent and used like garden wire. To our knowledge, Betty had
never experienced wire or similarly pliant objects whilst in captivity,
apart from 1 hour of free manipulation with flexible pipe-cleaners a
year before this experiment (which she was not seen to bend or use as
tools).
In subsequent experiments,
Betty was presented with the same apparatus but a new material for tool
making: flat strips of aluminium. On most trials, she first attempted to
retrieve the bucket with the unmodified material, but the duration of
these attempted probes declined rapidly (the median duration was just 3
seconds). After these attempts with the straight strips, in the first
few trials she attempted to make distal bends, following her previous
techniques (Weir & Kacelnik, 2006), but because of the properties of the
new material she was unsuccessful. (The strips could not be wedged in a
substrate in the same manner as the wire.) Betty then developed a new
technique, proximal bending, that was more effective with the aluminium
and which she used on all subsequent occasions (Figure 12a;
Movie 7).
This involved bending the end of the strip that was held in her beak,
rather than the distal end as previously. A consequence of this
technique was that after modification she was holding the modified end
of the tool, so the instrument needed to be turned around before it
could be used. However, in 5 of the first 10 valid trials, she initially
attempted to retrieve the bucket with the unmodified end of the strip
whilst holding the hooked end.
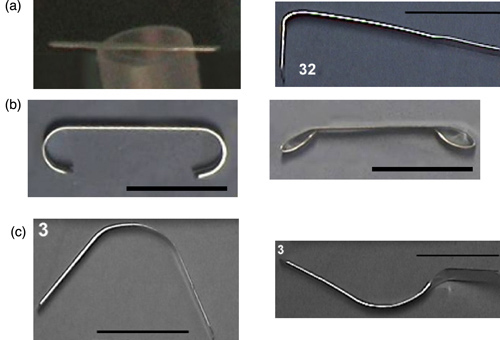
Figure 12. Tools used by a
captive crow (Betty) during experiments where she had to
(a) make hooks (by bending the tool); (b) make the tool
narrower (by squeezing or unbending it); or (c) make the
tool longer (by unbending it) to retrieve food (Weir &
Kacelnik, 2006). The original tools supplied are on the
left, and the corresponding tools after being modified
by her are on the right. Scale bar is 3 cm. Photos: Alex
Weir (reprinted with permission). |
|
Interpreting the
significance of Betty’s behaviour in terms of causal understanding is
not straightforward. One approach is to ask what behaviour we would
expect from an agent capable solely of trial-and-error learning. Such an
agent would presumably have learned in the first experiment (Weir et
al., 2002) that a certain sequence of actions with the wire leads to
success: First, the distal end of the wire must be wedged, then the
proximal end pulled at an angle at the distal end, then the wire removed
and inserted into the tube and used to pull up the bucket. Since the new
task and material closely resembled the old one, we would expect such an
agent to perform initially the same series of actions—which is indeed
what we observed with Betty. Following failure of this sequence, the
agent’s behaviour should become more variable (since variability
increases in extinction: e.g., Neuringer, Kornell, & Olufs, 2001), but
in a (relatively) random fashion. This was not what happened with Betty:
Her behaviour did become more variable, but it focussed specifically
upon manipulating the shape of the tool, rather than on other components
of the previously-successful sequence, such as the tool insertion or
lifting action.
Therefore, it seems that
Betty’s bending actions were to some extent goal directed in that she
was intent on producing a hook or hook-like modification. It also
appears that she had some understanding of how to make a hook, since she
very quickly converged on an efficient, novel way of doing so. In other
words, in both experiments she was able to invent a method of shaping
the material into a functional and probably preconceived form. However,
these results suffer from the problem raised above: namely, that it is
inherently difficult to pose a null hypothesis against which to test
these results. We are, therefore, unable to quantify the statistical
significance of our observations, highlighting a fundamental problem in
the investigation of creative behaviour.
Betty’s repeated attempts
to use the wrong end of the modified strips suggest that she did not
understand exactly why a hook was needed— certainly, an agent who
fully understood the task should never probe with the wrong end of the
tool. However, we should be cautious about leaping to judgements of
intellectual inability based on inefficient performance since humans do
sometimes make similar mistakes despite undoubtedly possessing
sophisticated folk physics (discussed in more detail later in this
section). It is also interesting to note that chimpanzees only correctly
reoriented a hooked tool on 6 of 28 trials (4 trials per subject, 7
subjects), which was the same as the number of times they reoriented a
straight tool (Povinelli, Reaux, Theall, & Giambrone, 2000b, Experiment
16 conditions E and G).
We tested Betty’s
understanding of the relationship between tool-shape and success with
two additional brief experiments involving more radical transformations
of the tasks (Weir & Kacelnik, 2006). In the first, the aluminium strip
was pre-bent at both ends, preventing it from being inserted through a
narrow hole—an action which was necessary to dislodge food. Betty did
modify the tool and obtain food on the only valid trial (in two trials
she managed to retrieve food without modifying the tool), although we
cannot conclude that this action was goal-directed. She flattened both
ends of the tool (Figure 12b), which may have been for ergonomic reasons
(ease of holding) rather than a purposeful modification of the ends to
serve two distinct functions (proximal end to be held in the beak,
distal one to fit the hole).
In the second experiment,
the aluminium strip was provided pre-bent into a broad U-shape, which
was too short for the task; it had to be lengthened by unbending to
retrieve food from a horizontal tube. Betty unbent the tool and obtained
the food on two of the three valid trials (again, Betty retrieved food
on one trial in a non-anticipated manner; Figure 12c;
Movie 8), although
on both occasions she first attempted to reach the food with the
unmodified tool. Sadly, Betty died before completing more replicates,
and the small number of trials together with the variability in her behaviour (to be expected in this kind of experiment) precludes
statistical analysis. However, it is important to note that she never
performed a bending action in these tasks, even though this had been the
behaviour consistently deployed and rewarded in previous experiments.
Thus, while her ability to ‘design by reasoning’ cannot be asserted, it
is reasonable to doubt a simple associative-learning account: In spite
of her reinforcement history, bending was not her generalised response
to inaccessible food and manipulable material (Figure 12).
Although Betty did not show
a human-level understanding of the causal need for a hook, her degree of
conceptualisation of the problem seems to exceed that previously
documented in other animals. Of particular relevance are a series of
tool-modification experiments with captive chimpanzees (Povinelli,
Reaux, Theall, & Giambrone, 2000a). In these studies, 7 chimpanzees were
each given 12 trials where they had to unbend a tool to retrieve the
reward, interspersed with 18 probe trials where no modification was
necessary. Only one subject (‘Jadine’) ever successfully unbent the tool
when it was necessary to do so, and she did so only on the final four
test trials following explicit training (to bend the tool). Moreover,
when only one end of the tool was bent, many of the subjects
preferentially attempted to insert the wrong end into the apparatus
throughout the testing period. These results suggest that chimpanzees
can learn (in this case through explicit provision of information) the
properties of tools while remaining ignorant of the abstract concepts
involved in successful tool use.

Figure 13. A human subject
(‘Bob’) attempts to replicate Betty’s wire-bending task
(see Figure 11). On the left Betty (mounted) illustrates
the original behaviour. Both Betty and Bob performed in
the presence of a conspecific (Abel and ‘Charles’
respectively). Photo: Alex Kacelnik (reprinted with
permission). |
|
What the experiments with
Betty do confirm is that understanding does not conform to a
presence/absence dichotomy. Some anecdotal observations with humans may
serve to illustrate this point. We recently presented our research on
New Caledonian crows at the Royal Society of London’s ‘Summer Science
Exhibition.’ We asked some visitors to our stall—adults and teenagers,
including many Fellows of the Royal Society and even its most recent
past President (Figure 13)—to participate in an informal experiment
resembling that conducted with Betty and Abel. Subjects were given the
opportunity to extract souvenir badges from a vertical, transparent
tube, using a pair of pliers (to simulate a crow’s beak) and a piece of
straight wire or aluminium strip. We explained that the goal of the task
was to extract the badge, and most visitors had seen video footage of
Betty’s successful wire bending. Surprisingly, and like Betty had done
during early trials, a substantial proportion of people attempted to
retrieve the reward without modifying the starting material—an approach
that was only successful in a small fraction of attempts. Many other
participants produced an exaggerated hook with a U-shaped tip that was
unsuitable for the desired task. In a few particularly revealing cases,
subjects bent a suitable hook—probably imitating Betty—but then inserted
the straight, non-functional end into the tube! This exercise had a
pedagogical and slightly humorous intention, and is hardly a rigorous
test of humans’ ability on this task. Yet, despite their definite
understanding of the goal as well as their likely knowledge of the
pliability of wire, many humans did not deduce the utility of a hook or
design it correctly before engaging in some practical testing. In humans
as in crows, it seems that performance on physical tasks may not always
be based on a strong form of a priori understanding, but that
understanding may in fact be promoted by some practical engagement with
the task itself (a similar point was made by Hunt, Rutledge, et al.,
2006).
Concluding Remarks
Betty’s manufacture of
hooks from novel materials and Guillem’s performance in the modified
trap-tube task are, to our knowledge, amongst the closest any non-humans
have come to demonstrating an ‘understanding’ of folk physics. However,
as we have mentioned earlier, the concept of understanding is an
epistemological minefield, and in what follows we examine at greater
length some of the issues raised by this word and their implications for
comparative cognition research.
In the earlier description
of Betty’s wire-bending behaviour, we stated that "she was able to
invent a method of shaping the material into a functional and probably
preconceived form." If ‘invent’ were exchanged for ‘discover’ and
‘preconceived’ were swapped for ‘previously learned,’ there would be no
need to look beyond associative learning and generalisation, albeit of a
relatively complex and unlikely nature. We chose the original wording
partly as a teaser (surely some readers’ reservations about
anthropomorphic language must have been aroused by the sentence) and
partly because, in our opinion, this possibility is worth entertaining
as a working hypothesis. We adhere to the spirit of Morgan’s Canon
(Thomas, 2001), and certainly do not argue that, because Betty acted as
a human might, she did so on the same conceptual basis (‘argument by
analogy’; see Povinelli, Bering, & Giambrone, 2000). However, to dismiss
the possibility of higher cognition out-of-hand could be construed as
anthropocentric in itself (Keeley, 2004). On the basis of the available
evidence, we cannot yet exclude the possibility that all apparently
intelligent behaviours shown by New Caledonian crows can be explained by
associative learning mechanisms, and the same may be true for other
non-human species. Given this situation, is it still meaningful or
useful to refer to understanding in crows and in animal behaviour in
general?
The term ‘understanding’
has been discussed in great depth by philosophers (e.g., Overskeid,
2005; Searle, 1980), and there is still no general agreement about how
it should be defined or how it can be conclusively demonstrated, even in
humans. We have mentioned Overkeid’s definition that "understanding X
means not believing lack of knowledge about X to be an obstacle to
reaching a relevant, currently active goal" (Overskeid, 2005, p. 601),
which implies that understanding is a default state, and most animals
(including people) "are probably in a state of understanding most of the
time" (p. 612). Overskeid generated this definition by analysing
situations in which humans would claim that they did or did not
understand something, a process which—while entirely valid from a
philosophical point of view—necessarily produces a definition from a
first-person perspective that is unsuitable for application in animal
cognition research. Overskeid proposes that we can judge understanding
in others by observing whether they behave appropriately in certain
situations; if so, we can ascribe to them some degree of understanding
in the relevant domain. For example, if someone anticipates rain and
prepares herself by carrying an umbrella, Overskeid would infer that she
had some understanding of meteorology. However, this definition takes no
account of the process by which the subject acquired proficiency in that
situation—if, for example, the subject evolved in an environment where
the weather could be predicted by simple, reliable cues, and she was
genetically endowed with rules that enabled accurate weather prediction,
it would seem inappropriate to ascribe any degree of understanding to
her, regardless of her successful performance (indeed, plants ‘predict’
the weather every spring when the days get longer). This definition also
says nothing about what
the subject understands or the correctness of her understanding.
In the domain of folk
physics, when we say that an animal ‘understands’ the problem, we
usually intend to mean that they have some (more-or-less correct)
knowledge of the causal basis of the task (Vonk, 2005). However, this
introduces new problems with what we mean by ‘knowledge’ and ‘causal
basis.’ Knowledge can be obtained in various ways, one of which is
associative learning, and there is no universal agreement on how to
separate associatively-learned
knowledge from associatively-learned behaviour. Equally, most
humans have only a limited, proximate understanding of the causal basis
of phenomena around them, including their actions. For instance, one can
say that the reason images disappeared from the TV screen is because the
set was unplugged, but this does not imply the converse: that the
subject understands why and how plugging the TV in causes the images to
be formed. Thus, a pertinent question is how accurate and detailed
causal understanding needs to be for us to recognise it as such. We do
not have a precise answer and remain in the quandary described in the
introduction: At the moment there is no definite test for understanding.
We believe, however, that progress is possible if one relaxes the
epistemological strictures just enough to foster empirical research,
while avoiding over-interpreting results. The role of theory in this
case resembles that of evolutionary assumptions in much of behavioural
ecology: While precise definitions of fitness remain a matter of debate
(Grafen, 1999), predictive models based on intuitive components of
fitness, such as foraging performance, continue to lead to significant
advances.
Our brief discussion of
philosophical matters testifies to our interest in the wider
implications of our research. New Caledonian crows and other corvids
exhibit behaviour that looks ‘smart’ to most human observers, and some
observations cry out for comparative work with other seemingly
intelligent animals, notably humans and other primates. Such comparisons
are important and have produced some striking examples of convergent
evolution in birds and mammals. Empirical evidence for corvid
intelligence is accumulating rapidly and has led some authors to propose
that "corvids should perhaps be considered as ‘feathered’ apes" (Emery,
2004, p. 182; see also Clayton & Emery, 2005). We naturally share the
general enthusiasm for corvid research but think that care needs to be
taken when interpreting and describing results. In particular, we need
to be cautious of ascribing general cognitive abilities to the whole
Corvidae family based on the abilities of individual species, which may
reflect adaptations to specific ecological niches. For example, western
scrub-jays and common ravens rely heavily on food caching and raiding
conspecifics’ caches, and elegant experimental investigations have
demonstrated that they are equipped with complex and flexible
cache-protection strategies and mechanisms (e.g., Bugnyar & Heinrich,
2005; Dally, Emery, & Clayton, 2005, 2006; Emery & Clayton, 2001).
Similarly, pinyon jays (Gymnorhinus cyanocephalus) are highly
social, and can use transitive reasoning to predict the dominance of
conspecifics (Paz-y-Miño C., Bond, Kamil, & Balda, 2004). Whilst it is
possible that these abilities are equivalent to the similar
cognitive processes in humans and are shared by the whole Corvidae
family, it could equally be the case that they are species-specific
adaptations. The remarkable tool-oriented behaviour of New Caledonian
crows may be another example of such an adaptation. In contrast, many of
these cognitive abilities appear to be found in all species of great ape
(Tomasello & Call, 1997) and are exhibited in a wide variety of
different contexts, making it more plausible that in apes, they are
truly the consequence of some kind of ‘general’ intelligence. However,
in many ways corvid research is still decades behind primate research,
particularly in terms of long-term field studies that are necessary for
putting experimental findings into an ecological and evolutionary
perspective, so it would be premature to reach firm conclusions at this
stage (a point also made by others, e.g., Emery, 2006; Emery & Clayton,
2004a).
We have recently attempted
to identify possible evolutionary origins of the behavioural action
patterns that lead to tool use in New Caledonian crows (Kenward et al.,
2007). Thomas Bugnyar and co-workers from Austria had documented in
detail the development of juvenile common ravens, a species that does
not habitually use tools in the wild, but is otherwise renowned for its
cognitive capacities (Bugnyar & Heinrich, 2005; Heinrich, 1999). Taking
advantage of the fact that the Austrian and the Oxford research groups
had employed similar (albeit not identical) observation protocols for
their longitudinal studies, we compared the ontogeny of object-oriented
behaviours between the two species. Our analyses revealed striking
developmental similarities between TOB in crows and food-caching
behaviour in ravens, including similar precursor behaviours. Given that
the common ancestor of New Caledonian crows and ravens was almost
certainly a caching species (de Kort & Clayton, 2006), we hypothesise
that the action patterns for tool use in crows have their evolutionary
origins in caching behaviour.
Tool use in New Caledonian
crows is the result of natural selection, yet we remain largely ignorant
of the selective forces that may have fostered this unusual behaviour in
the evolutionary past and those that maintain it under present-day
conditions. In 2005 we launched a long-term research project to study
New Caledonian crows in the wild. It is our hope that our observations
in the field, together with those of our colleagues from New Zealand,
will inform experimental work with captive crows. This concerted work in
the lab and in the field may yield answers to the many questions we were
unable to address in this review.
References
Alcock, J. (1972). The evolution of the use of tools by feeding animals.
Evolution, 26, 464-473.
Amsel, E., Goodman, G., Savoie, D., & Clark, M. (1996). The development of
reasoning about causal and noncausal influences on levers. Child Development,
67, 1624-1646.
Anderson, J. R., & Henneman, M. C. (1994). Solutions to a tool-use problem in a
pair of Cebus apella.
Mammalia, 58, 351-361.
Andersson, S. (1989). Tool use by the fan-tailed raven (Corvus rhipidurus).
Condor, 91, 999.
Barnard, C. J. (2004). Animal behaviour: Mechanism, development, function and
evolution. Harlow: Prentice Hall.
Beck, B. B. (1980). Animal tool behavior: The use and manufacture of tools by
animals. New York, NY: Garland.
Beck, B. B. (1986). Tools and intelligence. In R. J. Hoage & L. Goldman (Eds.),
Animal intelligence: Insights into the animal mind (pp. 135-147). Washington
D.C.: Smithsonian Institution Press.
Biro, D., Inoue-Nakamura, N., Tonooka, R., Yamakoshi, G., Sousa, C., &
Matsuzawa, T. (2003). Cultural innovation and transmission of tool use in wild
chimpanzees: Evidence from field experiments. Animal Cognition, 6,
213-223.
Blaisdell, A. P., Sawa, K., Leising, K. J., & Waldmann, M. R. (2006). Causal
reasoning in rats. Science, 311, 1020-1022.
Boesch, C., & Boesch, H. (1990). Tool use and tool making in wild chimpanzees.
Folia Primatologica, 54, 86-99.
Boesch, C., & Boesch, H. (1993). Diversity of tool use and tool-making in wild
chimpanzees. In A. Berthelet & J. Chevaillon (Eds.), The use of tools by
human and non-human primates (pp. 158-187). Oxford: Clarendon Press.
Borsari, A., & Ottoni, E. B. (2005). Preliminary observations of tool use in
captive hyacinth macaws (Anodorhynchus hyacinthinus). Animal
Cognition, 8, 48-52.
Boswall, J. (1978). Further notes on tool using by birds and related behaviour.
Avicultural Magazine, 84, 162-166.
Boswall, J. (1983). Tool-using and related behaviour by birds: More notes.
Avicultural Magazine, 89, 94-108.
Boyd, R., & Silk, J. B. (2006). How humans evolved (4th ed.). New York:
W.W. Norton.
Bugnyar, T., & Heinrich, B. (2005). Ravens, Corvus corax, differentiate between
knowledgeable and ignorant competitors. Proceedings of the Royal Society of
London B, 272, 1641-1646.
Caffrey, C. (2000). Tool modification and use by an American Crow. Wilson
Bulletin, 112, 283-284.
Call, J. (2004). Inferences about the location of food in the great apes (Pan
paniscus, Pan troglodytes,
Gorilla gorilla, and Pongo pygmaeus). Journal of
Comparative Psychology, 118, 232-241.
Chappell, J. (2006). Avian cognition: Understanding tool use. Current
Biology, 16, R244-R245.
Chappell, J., & Kacelnik, A. (2002). Tool selectivity in a non-mammal, the New
Caledonian crow (Corvus moneduloides). Animal Cognition, 5, 71-78.
Chappell, J., & Kacelnik, A. (2004). Selection of tool diameter by New
Caledonian crows Corvus moneduloides.
Animal Cognition, 7, 121-127.
Chappell, J., & Kacelnik, A. (2007). Folk physics in New Caledonian crows: Do
crows understand gravity? Manuscript in preparation.
Clayton, N. S. (1992). The ontogeny of food-storing and retrieval in marsh tits.
Behaviour, 122, 11-25.
Clayton, N. S., & Emery, N. J. (2005). Corvid cognition. Current Biology, 15,
R80-R81.
Cole, P. D. (2004). The ontogenesis of innovative tool use in an American
Crow (Corvus brachyrhynchos). Unpublished doctoral dissertation, Dalhousie
University, Canada, Halifax, Nova Scotia.
Collias, E. C., & Collias, N. E. (1964). The development of nest-building
behaviour in a weaverbird. Auk, 81, 42-52.
Cristol, D. A., & Switzer, P. V. (1999). Avian prey-dropping behavior. II.
American crows and walnuts.
Behavioral Ecology, 10, 220-226.
Dally, J. M., Emery, N. J., & Clayton, N. S. (2005). Cache protection strategies
by western scrub-jays,
Aphelocoma californica: Implications for social cognition.
Animal Behaviour, 70, 1251-1263.
Dally, J. M., Emery, N. J., & Clayton, N. S. (2006). Food-caching Western
scrub-jays keep track of who was watching when. Science, 312, 1662-1665.
de Houwer, J., Beckers, T., & Vandorpe, S. (2005). Evidence for the role of
higher order reasoning processes in cue competition and other learning
phenomena. Learning & Behavior, 33, 239-249.
de Kort, S. R., & Clayton, N. S. (2006). An evolutionary perspective on caching
by corvids. Proceedings of the Royal Society of London B, 273, 417-423.
Délacour, J. (1966). Guide des oiseaux de la Nouvelle-Calédonie et de ses
dépendances. Paris: Delachaux et Niestlé.
Dickinson, A. (2001). Causal learning: Association versus computation.
Current Directions in Psychological Science, 10, 127-132.
Emery, N. J. (2004). Are corvids ‘feathered apes’? Cognitive evolution in crows,
jays, rooks and jackdaws. In S. Watanabe (Ed.), Comparative analysis of minds
(pp. 181-213). Tokyo: Keio University Press.
Emery, N. J. (2006). Cognitive ornithology: The evolution of avian intelligence.
Philosophical Transactions of the Royal Society of London B, 361, 23-43.
Emery, N. J., & Clayton, N. S. (2001). Effects of experience and social context
on prospective caching strategies by scrub jays. Nature, 414, 443-446.
Emery, N. J., & Clayton, N. S. (2004a). Comparing the complex cognition of birds
and primates. In L. J. Rogers & G. Kaplan (Eds.), Comparative vertebrate
cognition: Are primates superior to non-primates? (pp. 3-55). New York:
Kluwer Academic / Plenum Publishers.
Emery, N. J., & Clayton, N. S. (2004b). The mentality of crows: Convergent
evolution of intelligence in corvids and apes. Science, 306, 1903-1907.
Fitch, W. T., Hauser, M. D., & Chomsky, N. (2005). The evolution of the language
faculty: Clarifications and implications. Cognition, 97, 179-210.
Fragaszy, D. M., & Adams-Curtis, L. E. (1997). Developmental changes in
manipulation in tufted capuchins (Cebus apella) from birth through 2
years and their relation to foraging and weaning. Journal of Comparative
Psychology, 111, 201-211.
Freeman, S. (2002). Biological science
(2nd ed.). Upper Saddle River, NJ: Pearson Prentice Hall.
Galef, B. G., Jr. (1988). Imitation in animals: History, definition, and
interpretation of data from the psychological laboratory. In T. R. Zentall & B.
G. Galef, Jr (Eds.),
Social learning: Psychological and biological perspectives
(pp. 3-28). Hillsdale, NJ: Lawrence Erlbaum Associates, Inc.
Gayou, D. C. (1982). Tool use by green jays.
Wilson Bulletin, 94, 593-594.
Gibson, E. J., & Pick, A. D. (2000). An ecological approach to perceptual
learning and development.
Oxford: Oxford University Press.
Grafen, A. (1999). Formal Darwinism: The individual-as-maximizing-agent analogy
and bet-hedging.
Proceedings of the Royal Society of London B, 266, 799-803.
Hall, K. R. L. (1963). Tool-using performances as indicators of behavioural
adaptability. Current Anthropology, 4, 479-494.
Hansell, M. H. (1984). Animal architecture and building behaviour.
London: Longman.
Hansell, M. H. (1987, January 8). What’s so special about using tools? New
Scientist, 1542, 55-56.
Hansell, M. H. (2005). Animal architecture. Oxford: Oxford University
Press.
Hauser, M. D. (1997). Artifactual kinds and functional design features: What a
primate understands without language. Cognition, 64, 285-308.
Hauser, M. D., Kralik, J., & Botto-Mahan, C. (1999). Problem solving and
functional design features: Experiments on cotton-top tamarins, Saguinus
oedipus oedipus. Animal Behaviour, 57, 565-582.
Hauser, M. D., Pearson, H., & Seelig, D. (2002). Ontogeny of tool use in
cottontop tamarins, Saguinus oedipus: Innate recognition of functionally
relevant features. Animal Behaviour, 64, 299-311.
Hauser, M. D., & Santos, L. R. (in press). The evolutionary ancestry of our
knowledge of tools: From percepts to concepts. In E. Margolis & S. Lawrence
(Eds.), Creations of the mind. Oxford, UK: Oxford University Press.
Hauser, M. D., Santos, L. R., Spaepen, G. M., & Pearson, H. E. (2002). Problem
solving, inhibition and domain-specific experience: Experiments on cottontop
tamarins,
Saguinus oedipus. Animal Behaviour, 64, 387-396.
Hayashi, M., & Matsuzawa, T. (2003). Cognitive development in object
manipulation by infant chimpanzees. Animal Cognition, 6, 225-233.
Heinrich, B. (1999). The mind of the raven: Investigations and adventures
with wolf-birds. New York: Cliff Street Books.
Henty, C. J. (1986). Development of snail-smashing by song thrushes. British
Birds, 79, 277-281.
Heyes, C. M. (1994). Social learning in animals: Categories and mechanisms.
Biological Reviews of the Cambridge Philosophical Society, 69, 207-231.
Hicks, T. C., Fouts, R. S., & Fouts, D. H. (2005). Chimpanzee (Pan
troglodytes troglodytes) tool use in the Ngotto Forest, Central African
Republic. American Journal of Primatology, 65, 221-237.
Hunt, G. R. (1996). Manufacture and use of hook-tools by New Caledonian crows.
Nature, 379, 249-251.
Hunt, G. R. (2000a). Human-like, population-level specialization in the
manufacture of pandanus tools by New Caledonian crows Corvus moneduloides.
Proceedings of the Royal Society of London B, 267, 403-413.
Hunt, G. R. (2000b). Tool use by the New Caledonian crow Corvus moneduloides
to obtain Cerambycidae from dead wood. Emu, 100, 109-114.
Hunt, G. R., Corballis, M. C., & Gray, R. D. (2001). Laterality in tool
manufacture by crows. Nature, 414, 707.
Hunt, G. R., Corballis, M. C., & Gray, R. D. (2006). Design complexity and
strength of laterality are correlated in New Caledonian crows’ pandanus tool
manufacture. Proceedings of the Royal Society of London B, 273,
1127-1133.
Hunt, G. R., & Gray, R. D. (2002). Species-wide manufacture of stick-type tools
by New Caledonian Crows. Emu, 102, 349-353.
Hunt, G. R., & Gray, R. D. (2003). Diversification and cumulative evolution in
New Caledonian crow tool manufacture. Proceedings of the Royal Society of
London B, 270, 867-874.
Hunt, G. R., & Gray, R. D. (2004a). The crafting of hook tools by wild New
Caledonian crows. Proceedings of the Royal Society of London B (Suppl.), 271,
S88-S90.
Hunt, G. R., & Gray, R. D. (2004b). Direct observations of pandanus-tool
manufacture and use by a New Caledonian crow (Corvus moneduloides).
Animal Cognition, 7, 114-120.
Hunt, G. R., Rutledge, R. B., & Gray, R. D. (2006). The right tool for the job:
What strategy do wild New Caledonian crows use? Animal Cognition, 9,
307-316.
Hunt, G. R., Sakuma, F., & Shibata, Y. (2002). New Caledonian crows drop
candle-nuts onto rock from communally-used forks on branches. Emu, 102,
283-290.
Jones, T., & Kamil, A. C. (1973). Tool-making and tool-using in the northern
blue jay. Science, 180, 1076-1078.
Kacelnik, A., Chappell, J., Weir, A. A. S., & Kenward, B. (2006). Cognitive
adaptations for tool-related behaviour in New Caledonian crows. In E. A.
Wasserman & T. R. Zentall (Eds.),
Comparative cognition: Experimental explorations of animal
intelligence (pp. 515-528). Oxford: Oxford University Press.
Keeley, B. L. (2004). Anthropomorphism, primatomorphism, mammalomorphism:
Understanding cross-species comparisons. Biology & Philosophy, 19,
521-540.
Kenward, B. (2006). Why do New Caledonian crows use tools? Unpublished
doctoral dissertation, University of Oxford, Oxford.
Kenward, B., Rutz, C., Weir, A. A. S., Chappell, J., & Kacelnik, A. (2004).
Morphology and sexual dimorphism of the New Caledonian Crow Corvus
moneduloides, with notes on its behaviour and ecology. Ibis, 146,
652-660.
Kenward, B., Rutz, C., Weir, A. A. S., & Kacelnik, A. (2006). Development of
tool use in New Caledonian crows: Inherited action patterns and social
influence. Animal Behaviour, 72, 1329-1343.
Kenward, B., Schlögl, C., Rutz, C., Weir, A. A. S., Bugnyar, T., & Kacelnik, A.
(2007). On the evolutionary origin of tool-oriented behaviour in New
Caledonian crows. Manuscript in preparation.
Kenward, B., Weir, A. A. S., Rutz, C., & Kacelnik, A. (2005). Tool manufacture
by naive juvenile crows.
Nature, 433, 121.
Laland, K. N., & Janik, V. M. (2006). The animal cultures debate. Trends in
Ecology & Evolution, 21, 542-547.
Layard, E. L., & Layard, E. L. C. (1882). Notes on the avifauna of New
Caledonia. Ibis, 6, 520-522.
Lefebvre, L., Reader, S. M., & Sol, D. (2004). Brains, innovations and evolution
in birds and primates. Brain, Behavior and Evolution, 63, 233-246.
Limongelli, L., Boysen, S. T., & Visalberghi, E. (1995). Comprehension of
cause-effect relations in a tool-using task by chimpanzees (Pan troglodytes).
Journal of Comparative Psychology, 109, 18-26.
Lockman, J. J. (2000). A perception-action perspective on tool use development.
Child Development, 71, 137-144.
Lonsdorf, E. V. (2005). Sex differences in the development of termite-fishing
skills in the wild chimpanzees, Pan troglodytes schweinfurthii, of Gombe
National Park, Tanzania.
Animal Behaviour, 70, 673-683.
Lonsdorf, E. V. (2006). What is the role of mothers in the acquisition of
termite-fishing behaviors in wild chimpanzees (Pan troglodytes schweinfurthii)?
Animal Cognition, 9, 36-46.
Machado, A., & Silva, F. J. (2003). You can lead an ape to a tool, but …: A
review of Povinelli’s Folk physics for apes: The chimpanzee’s theory of how the
world works. Journal of the Experimental Analysis of Behavior, 79,
267-286.
Marler, P., & Slabbekoorn, H. (Eds.). (2004).
Nature’s music: The science of birdsong. Amsterdam: Elsevier
Academic Press.
Mulcahy, N. J., Call, J., & Dunbar, R. I. M. (2005). Gorillas (Gorilla
gorilla) and orangutans (Pongo pygmaeus) encode relevant problem
features in a tool-using task.
Journal of Comparative Psychology, 119, 23-32.
Neuringer, A., Kornell, N., & Olufs, M. (2001). Stability and variability in
extinction. Journal of Experimental Psychology: Animal Behavior Processes, 27,
79-94.
Nishida, T. (1972). The ant-gathering behaviour by the use of tools among wild
chimpanzees of the Mahali Mountains.
Journal of Human Evolution, 2, 357-370.
Orenstein, R. (1972). Tool use by the New Caledonian crow (Corvus
moneduloides). Auk, 89, 674-676.
Overskeid, G. (2005). Empirically understanding understanding can make problems
go away: The case of the Chinese room. Psychological Record, 55, 595-617.
Parker, S. T., & Gibson, K. R. (1977). Object manipulation, tool use and
sensorimotor intelligence as feeding adaptations in Cebus monkeys and great
apes. Journal of Human Evolution, 6, 623-641.
Paz-y-Miño C., G., Bond, A. B., Kamil, A. C., & Balda, R. P. (2004). Pinyon jays
use transitive inference to predict social dominance. Nature, 430,
778-781.
Penn, D. C., & Povinelli, D. J. (2007). Causal cognition in human and nonhuman
animals: A comparative, critical review. Annual Review of Psychology, 58,
97-118.
Pouydebat, E., Berge, C., Gorce, P., & Coppens, Y. (2005). Use and manufacture
of tools to extract food by captive
Gorilla gorilla gorilla: Experimental approach. Folia
Primatologica, 76, 180-183.
Povinelli, D. J. (Ed.). (2000). Folk physics for apes. Oxford, UK: Oxford
University Press.
Povinelli, D. J., Bering, J. M., & Giambrone, S. (2000). Toward a science of
other minds: Escaping the argument by analogy. Cognitive Science, 24,
509-541.
Povinelli, D. J., Reaux, J. E., Theall, L. A., & Giambrone, S. (2000a). The
question of tool modification. In D. J. Povinelli (Ed.), Folk physics for
apes (pp. 271-296). Oxford, UK: Oxford University Press.
Povinelli, D. J., Reaux, J. E., Theall, L. A., & Giambrone, S. (2000b). The
rope, hook, touching-stick, and related problems: The question of physical
connection. In D. J. Povinelli (Ed.), Folk physics for apes (pp.
206-253). Oxford, UK: Oxford University Press.
Reader, S. M., Sol, D., & Lefebvre, L. (2005). Comparing cognition across
species. Trends in Cognitive Sciences, 9, 411.
Reaux, J. E., & Povinelli, D. J. (2000). The trap-tube problem. In D. J.
Povinelli (Ed.), Folk physics for apes (pp. 108-131). Oxford, UK: Oxford
University Press.
Reid, J. B. (1982). Tool-use by a rook (Corvus frugilegus), and its causation.
Animal Behaviour, 30, 1212-1216.
Roth, G., & Dicke, U. (2005). Evolution of the brain and intelligence. Trends
in Cognitive Sciences, 9, 250-257.
Rutledge, R., & Hunt, G. R. (2004). Lateralized tool use in wild New Caledonian
crows. Animal Behaviour, 67, 327-332.
Santos, L. R., Miller, C. T., & Hauser, M. D. (2003). Representing tools: How
two non-human primate species distinguish between the functionally relevant and
irrelevant features of a tool. Animal Cognition, 6, 269-281.
Santos, L. R., Pearson, H., Spaepen, G. M., Tsao, F., & Hauser, M. (2006).
Probing the limits of tool competence: Experiments with two non-tool-using
species (Cercopithecus aethiops and Saguinus oedipus). Animal
Cognition, 9, 94-109.
Santos, L. R., Rosati, A., Sproul, C., Spaulding, B., & Hauser, M. D. (2005).
Means-means-end tool choice in cotton-top tamarins (Saguinus oedipus):
Finding the limits on primates’ knowledge of tools. Animal Cognition, 8,
236-246.
Sarsby, J. (Writer). (1998). The insatiable appetite [Television series
episode]. In M. Salisbury (Producer),
The life of birds. UK: BBC Natural History Unit.
Searle, J. R. (1980). Minds, brains, and programs. Behavioral and Brain
Sciences, 3, 417-425.
Seed, A. M., Tebbich, S., Emery, N. J., & Clayton, N. S. (2006). Investigating
physical cognition in rooks, Corvus frugilegus. Current Biology, 16,
697-701.
Silva, F. J., Page, D. M., & Silva, K. M. (2005). Methodological-conceptual
problems in the study of chimpanzees’ folk physics: How studies with adult
humans can help. Learning & Behavior, 33, 47-58.
Spaulding, B., & Hauser, M. D. (2005). What experience is required for acquiring
tool competence? Experiments with two Callitrichids. Animal Behaviour, 70,
517-526.
Tebbich, S., & Bshary, R. (2004). Cognitive abilities related to tool use in the
woodpecker finch, Cactospiza pallida. Animal Behaviour, 67,
689-697.
Tebbich, S., Seed, A. M., Emery, N. J., & Clayton, N. S. (in press).
Non-tool-using rooks (Corvus frigilegus) solve the trap-tube task.
Animal Cognition.
Tebbich, S., Taborsky, M., Fessl, B., & Blomqvist, D. (2001). Do woodpecker
finches acquire tool-use by social learning? Proceedings of the Royal Society
of London B, 268, 2189-2193.
Tebbich, S., Taborsky, M., Fessl, B., Dvorak, M., & Winkler, H. (2004). Feeding
behavior of four arboreal Darwin’s finches: Adaptations to spatial and seasonal
variability. Condor, 106, 95-105.
Thomas, R. K. (2001). Lloyd Morgan’s Canon: A history of misrepresentation.
History & Theory of Psychology Eprint Archive, 2006. Retrieved 26 September,
2006, from http://htpprints.yorku.ca/archive/00000017/
Thorndike, E. L. (1898). Animal intelligence: An experimental study of the
associative processes in animals.
Psychological Monographs, 2, 1-109.
Thouless, C. R., Fanshawe, J. H., & Bertram, B. C. R. (1989). Egyptian vultures
Neophron percnopterus and ostrich Struthio camelus eggs: The origins
of stone-throwing behavior. Ibis, 131, 9-15.
Tomasello, M., & Call, J. (1997). Primate cognition. New York, NY: Oxford
University Press.
Townsend, M. (2005, March 6). Sheep might be dumb ... but they’re not stupid.
The Observer, p. 10.
Ueno, Y., & Fujita, K. (1998). Spontaneous tool use by a Tonkean macaque (Macaca
tonkeana). Folia Primatologica, 69, 318-324.
Vargha-Khadem, F., Gadian, D. G., Copp, A., & Mishkin, M. (2005). FOXP2 and the
neuroanatomy of speech and language. Nature Reviews Neuroscience, 6,
131-138.
Visalberghi, E. (1993). Tool use in a South American monkey species: An overview
of the characteristics and limits of tool use in Cebus apella. In A.
Berthelet & J. Chevaillon (Eds.), The use of tools by human and non-human
primates (pp. 118-135). Oxford: Clarendon Press.
Visalberghi, E. (1997). Success and understanding in cognitive tasks: A
comparison between Cebus apella and
Pan troglodytes. International Journal of Primatology, 18,
811-830.
Visalberghi, E., Fragaszy, D. M., & Savage-Rumbaugh, E. S. (1995). Performance
in a tool-using task by common chimpanzees (Pan troglodytes), bonobos (Pan
paniscus), an orangutan (Pongo pygmaeus), and capuchin monkeys (Cebus
apella). Journal of Comparative Psychology, 109, 52-60.
Visalberghi, E., & Limongelli, L. (1994). Lack of comprehension of cause-effect
relations in tool-using capuchin monkeys (Cebus apella). Journal of
Comparative Psychology, 108, 15-22.
Visalberghi, E., & Trinca, L. (1989). Tool use in capuchin monkeys:
Distinguishing between performing and understanding. Primates, 30,
511-521.
Vonk, J. (2005). Causality in non-humans. In A. Reboul & G. Origgi (Eds.),
Causality. Institute for Cognitive Science and the University of Geneva.
Weir, A. A. S. (2006). Cognitive psychology of tool use in New Caledonian
crows (Corvus moneduloides). Unpublished doctoral dissertation, University
of Oxford, Oxford.
Weir, A. A. S., Chappell, J., & Kacelnik, A. (2002). Shaping of hooks in New
Caledonian crows. Science, 297, 981.
Weir, A. A. S., & Kacelnik, A. (2006). A New Caledonian crow (Corvus
moneduloides) creatively re-designs tools by bending or unbending aluminium
strips. Animal Cognition, 9, 317-334.
Wolpert, L. (2003). Causal belief and the origins of technology.
Philosophical Transactions of the Royal Society of London A, 361, 1709
-1719.
|